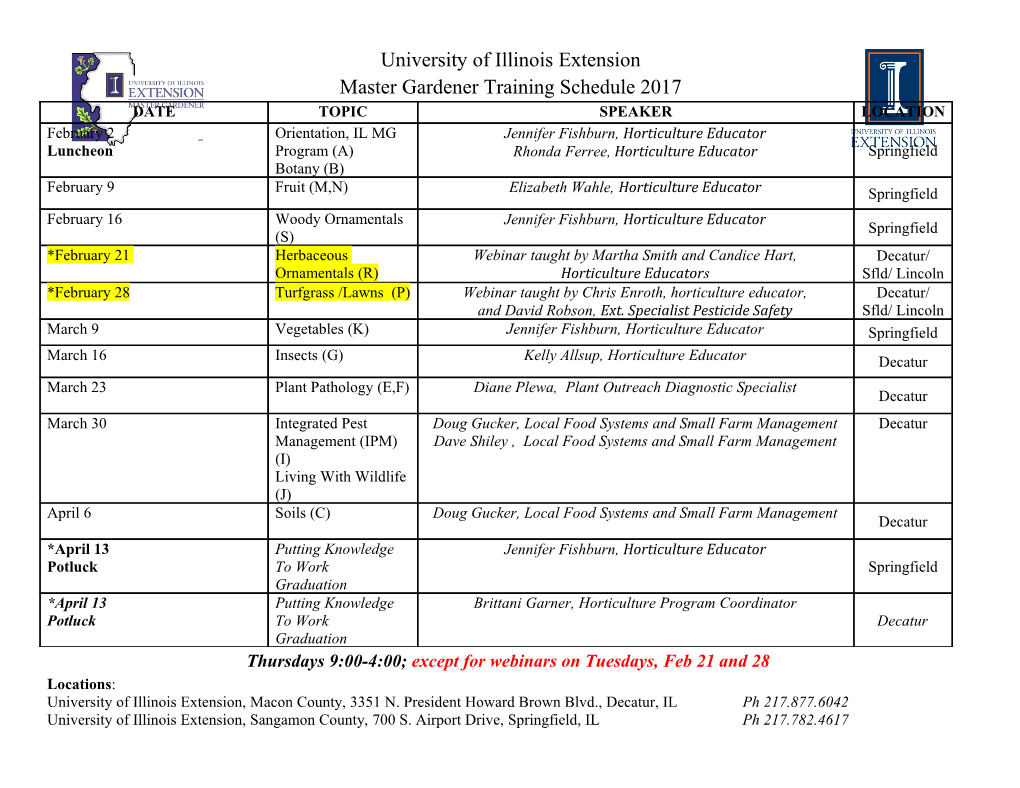
DOE FINAL TECHNICAL REPORT ON PHASE 1 1 PHASE I FINAL TECHNICAL REPORT FOR CONTRACT # DE-AC26-99FT40692 For the period of 10/01/99-09/30/00 With Stanford University. MULTI-ATTRIBUTE SEISMIC/ROCK PHYSICS APPROACH TO CHARACTERIZING FRACTURED RESERVOIRS Prepared for U. S. Department of Energy Federal Energy Technology Center by Gary Mavko,PI Stanford Rock Physics Project, Geophysics Department 397 Panama Mall, Mitchell 313, Stanford, CA 94305-2215 Phone: 650 723 9438; Fax: 650.723.1188 [email protected] October, 2000 DOE FINAL TECHNICAL REPORT ON PHASE 2 TABLE OF CONTENTS JUSTIFICATION…………………………………………………………PAGE 3. INTRODUCTION………………………………………………………...PAGE 5. SUMMARY OF PHASE I RESULTS………………………..…………..PAGE 6. PLANS FOR PHASE II…………………………………………………..PAGE 9. ATTACHMENTS: A. Fracture rock physics handbook. B. Geologic model of fracture occurrence in the Yates field. C. Rock physics and fracture modeling of the San Andres reservoir. D. Seismic detection of pore fluids: Pitfalls of ignoring anisotropy. DOE FINAL TECHNICAL REPORT ON PHASE 3 JUSTIFICATION During Phase I, we performed our rock physics, geologic, and seismic feasibility analysis for fracture characterization. We addressed the problem of seismic fracture detection and characterization, in general, and specifically at our field site in West Texas. In this analysis, we were able to implement a number of fracture modeling procedures and to quantitatively assess the theoretical detectability of reservoir fractures. This allowed us to identify which of the several seismic attributes are most likely to be useful for fracture detection at the site. One of our results showed that Thomsen’s parameters should be very useful for indicating gas-filled fractures at the site. We also outlined Monte Carlo approaches that can be applied for feasibility analysis at any site. Prior to our study, most seismic fracture detection methods have focused on qualitatively detecting seismic anisotropy. While anisotropy is critical, when used alone it has left us with interpretation ambiguities and nonuniqueness. During Phase 1 of this project, we found that by statistically integrating geologic and seismic information we can develop nontraditional methods for fracture detection. For example, we found that quantifying the correlations between fracture occurrence and depositional environment, and between fracture spacing and layer thickness, we allow us to decrease the uncertainty of fracture interpretation using seismic anisotropy. One of the reasons for this is that different depositional environments (and rock facies) give rise to differences in their seismic signatures (impedances, velocities, and Poisson’s ratio. Phase II will allow us to validate and improve these methodologies by carrying out a small- scale field pilot study. Taking methods to the field, always allows us to make them more applicable. In the field, we will be able to more accurately assess the uncertainties introduced by measurement noise, source and receiver response, coupling, and near surface acoustic properties. We can then more realistically develop ways to reduce the uncertainty, as well as to identify which aspects of the geology and fracture distribution we can characterize most robustly. The small-scale field study will also help us to establish empirical fracture parameters that are needed as inputs to rock physics models. For example, ALL of the effective medium models for fracture-induced anisotropy require fracture stiffness as inputs (these are sometimes characterized as moduli or aspect ratios). There is no realistic way to assign these values DOE FINAL TECHNICAL REPORT ON PHASE 4 theoretically, so being able to calibrate them in a small pilot will give us the critical inputs we need for interpreting the full scale seismic survey in phase III. Finally, the data from the pilot will allow us to add an aspect of "data mining" to the study. In Phase I, we have exploited our best theoretical tools, but the real field data will give us the opportunity to discover other, unpredicted, signatures of fractures that might prove valuable. Our accomplishments in Phase I suggest that Phase II will lead to fruitful and practical next generation fracture characterization methods. The practical outcome from Phase I is a methodology for modeling the seismic velocity, impedance, and reflectivity signatures of fractured reservoirs, based on well logs and the geologic data for any site of interest. The first part of this methodology is characterizing the natural variability of porosity and acoustic properties of the unfractured (matrix rock). The second part of the methodology is the means to explore how the geophysical signatures will change when fractures are added to that matrix. The most important contribution of the Phase I work is an approach that any explorationist can use when assessing whether fractures will be detectable, and what the uncertainty or risk of that interpretation will be. The seismic signatures are almost always non-unique and often non-distinct. Our phase I methodology quantifies this uncertainty and allows estimates to be made about how good the seismic experiment, signal/noise etc. has to be before it would be of any use for fracture interpretation. If an explorationist applied our methodology, he/she could use the results to make decisions about what sort of seismic experiment would be most likely to lead to successful fracture characterization. The results from such analyses could be used as inputs in other financial and economic analyses to decide how much money to spend on various seismic experiments. In many cases, the seismic measurement may not be able to 'image' the fracture directly, but the presence of fractures will give rise to various indirect seismic signatures that can be used in combination with geologic information to make interpretations about the fractures. Tech-transfer and dissemination of our research results to the industry is an integral part of our efforts. Our industry partner on this project, Marathon Oil, will have access to the new methodologies coming out this project. We will publish our results in professional journals and present them at international conferences. This plays a role in informing other explorationists about new methods. From time to time, we also give industry courses, which incorporate our recent research results, thus facilitating tech-transfer. DOE FINAL TECHNICAL REPORT ON PHASE 5 We anticipate that the practical outcome of Phase II will be (1) validation and improvement of the modeling methodology from Phase I, and (2) development of methodologies that can be applied specifically to the processing and interpretation of field seismic data for characterizing fractured reservoirs. We expect that the outcome will be more reliable and realistic interpretation of seismic and geologic data for fracture detection and mapping. INTRODUCTION This project consists of three key interrelated Phases, each focusing on the central issue of imaging and quantifying fractured reservoirs, through improved integration of the principles of rock physics, geology, and seismic wave propagation. This report summarizes the results of Phase I of the project. The key to successful development of low permeability reservoirs lies in reliably characterizing fractures. Fractures play a crucial role in controlling almost all of the fluid transport in tight reservoirs. Current seismic methods to characterize fractures depend on various anisotropic wave propagation signatures that can arise from aligned fractures. We are pursuing an integrated study that relates to high-resolution seismic images of natural fractures to the rock parameters that control the storage and mobility of fluids. Our goal is to go beyond the current state-of-the art to develop and demonstrate next generation methodologies for detecting and quantitatively characterizing fracture zones using seismic measurements. Our study incorporates 3 key elements: (1) Theoretical rock physics studies of the anisotropic viscoelastic signatures of fractured rocks, including up scaling analysis and rock-fluid interactions to define the factors relating fractures in the lab and in the field; (2) Modeling of optimal seismic attributes, including offset and azimuth dependence of travel time, amplitude, impedance and spectral signatures of anisotropic fractured rocks. We will quantify the information content of combinations of seismic attributes, and the impact of multi- attribute analyses in reducing uncertainty in fracture interpretations. (3) Integration and interpretation of seismic, well log, and laboratory data, incorporating field geologic fracture characterization and the theoretical results of items 1 and 2 above. The focal point for this project is the demonstration of these methodologies in the Marathon Oil Company Yates Field in West Texas. DOE FINAL TECHNICAL REPORT ON PHASE 6 SUMMARY OF PHASE I RESULTS The focus of Phase I research was the basic rock physics of fractured rocks, in particular the rock physics appropriate to the selected field site. Our Phase I objective was to establish the optimum seismic-to-rock properties transforms that relate observable seismic signatures – velocities, velocity dispersion, velocity anisotropy – to the rock, fracture, and pore fluid characteristics that control hydrocarbon storage and mobility. During this Phase, we worked on several activities focused on establishing, refining, and understanding the basic relations linking realistic fracture and fluid parameters with their geophysical
Details
-
File Typepdf
-
Upload Time-
-
Content LanguagesEnglish
-
Upload UserAnonymous/Not logged-in
-
File Pages160 Page
-
File Size-