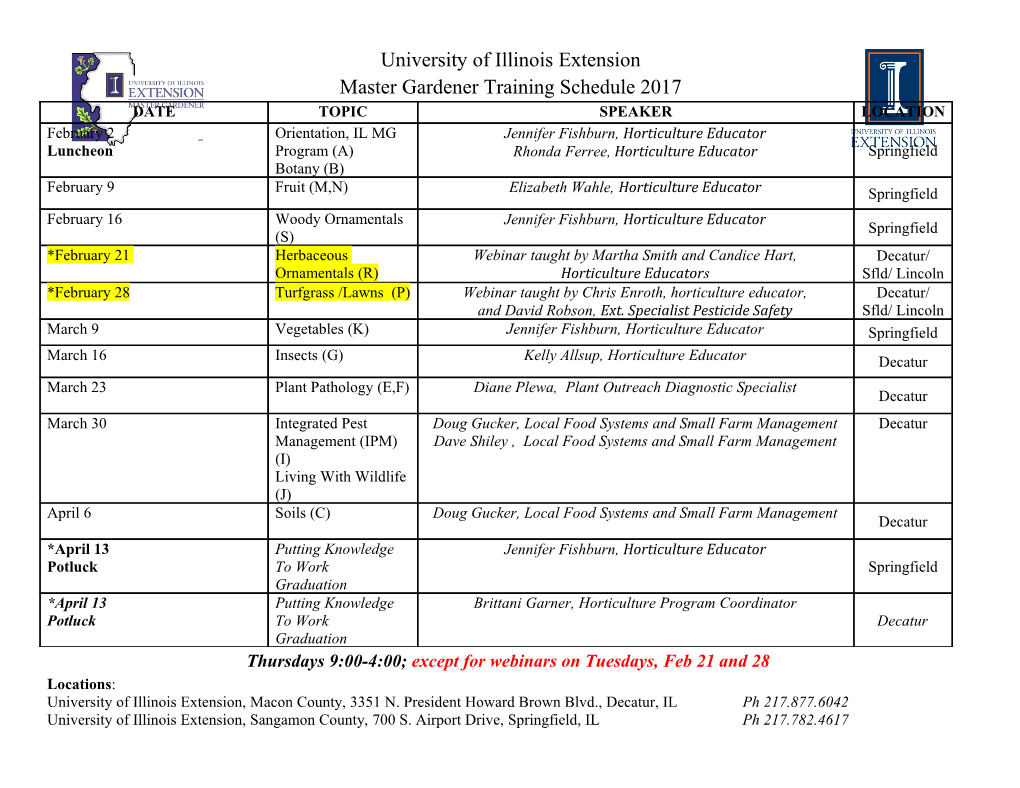
4A.2 OBSERVATIONS OF WALL CLOUD FORMATION IN SUPERCELL THUNDERSTORMS DURING VORTEX2 Nolan T. Atkins, Eva M. Glidden, and Timothy M. Nicholson Lyndon State College, Lyndonville, Vermont 05851 1. INTRODUCTION formation is based on idealized modeling results of Rotunno and Klemp (1985) and visual observations. The wall cloud is a lowering of cloud base In their idealized simulation of a supercell, Rotunno associated with the updraft of a thunderstorm. The and Klemp (1985) showed that the air entering the focus of this study concerns wall clouds formed storms updraft region where the wall cloud formed within supercell thunderstorms (Bluestein 1983; was being ingested from behind the gust front. This Davies-Jones 1986; Bluestein 1993). Early obser- rain-cooled air had descended from mid levels with- vational studies suggested that the supercell wall in the storm and subsequently saturated below the cloud is the visual indicator of a strong updraft core primary cloud base as it ascended with the low-level and may exhibit cyclonic rotation. (Moller et al. 1978; updraft that was dynamically forced by an upward Bluestein 1984). Recent studies have also revealed directed pressure gradient. Observations by storm the existence of anticyclonic wall clouds (Atkins et intercept teams have since confirmed that cloud al. 2012). National Weather Service storm spot- tags associated with cooler air behind the gust front ter training documents indicate that persistent wall often rise into the updraft. Often this occurs near or clouds that develop strong rotation and exhibit rapid in association with the distinct “tail cloud” that pro- upward vertical motion are often regarded as pre- trudes from near the base of the wall cloud toward cursors to tornadogenesis (http://www.nws.noaa. the cooler air. This mechanism is described in Na- gov/om/brochures/adv_spotters.pdf). While many tional Weather Service storm spotter training doc- studies of supercell thunderstorms have photo-doc- uments (http://www.nws.noaa.gov/training/wxspot. umented the wall cloud, amazingly little is known php). about their formation. To date, no observational study has been Our current understanding of wall cloud published confirming the Rotunno and Klemp (1985) 5 June, 2009 Photo Time: 2157:45 UTC (a) 5° Cloud Base ≈ 1100 m ARL 3° Wall Cloud RFD 900 m parcel Mesonet estimated wall cloud base: 530 m ±50 m FF 600 m parcels 1° 300 m 272° 274° 276° 277.4° 278° 280° 282° 284° 286° 288° 290° 292° FIG 1. Photograph of the wall cloud taken at 2157:45 UTC on 5 June 2009. Photogrammetric estimates of cloud base height and wall cloud vertical extent are shown in black. Mobile mesonet estimated wall cloud base height is shown in purple. Wall cloud boundary is shown in red. ___________ Corresponding author address: Nolan T. Atkins, Department of Atmospheric Sciences, Lyndon State College, Lyndonville, VT 05851 E-mail: [email protected] 1 5 June, 2009 2156 - 2158 UTC z = 600 m -1 Reflectivity (dBZ) W (ms ) -1 horizontal winds p’ (mb) 1 280° fr om CAMA location of minimum OW 277.4° 275° -6 approximate -3 wall cloud location -1 0 mb 1 4 km (a) 30 ms -1 (b) FIG 2.0 Dual-Doppler 5 10 15 20 data 25 dBZfrom 2156:0035 40 45 – 2158:00 50 55 UTC at -20 600 -15m ARL -10 is shown.-5 0 ms (a)-1 Radar 10 reflectivity 15 20 from DOW 7 (color) and dual-Doppler winds (ms-1; black vectors). Cyan lines are the left, center, and right azi- muths of the estimated wall cloud location based on photogrammetry. The thick red line is the approximate wall cloud location. The location of minimum Okubo-Weiss number is filled purple. (b) Vertical velocity (ms-1; color) and perturbation pressure (black contours) are shown. Wall cloud location and azimuths are same as in (a). mechanism for wall cloud formation. It is not known lect observations in and around supercell thunder- what fraction of air creating observed wall clouds storms. To increase the number of storm intercepts, originates in the storm mid levels or from other the experiment was mobile covering the South- source regions such as the inflow. Given that some ern, Central, and Northern Plains states during the wall clouds may be collocated with the low-level me- spring seasons of 2009 and 2010. More information socyclone and therefore contain significant low-level on VORTEX2 can be found in the review article by rotation, it is possible that some or all of the wall Wurman et al. (2012). cloud lowering is created by the adiabatic cooling Analyses of two supercells observed during associated with the pressure deficit at the circula- VORTEX2 are presented herein. The first formed tion center. While this mechanism may not explain on 5 June 2009 over Goshen CO Wyoming (here- all of the lowering for wall clouds associated with after referred to as the Goshen County supercell). weak rotation, it may explain a significant portion of This supercell produced a well-defined wall cloud the lowering associated with strongly rotating wall and attendant EF-2 tornado. The second supercell clouds. No study has systematically examined this was observed on 11-12 June 2009 west-northwest wall cloud formation mechanism. of La Junta CO (hereafter referred to as the La Junta supercell). While this supercell was tornado warned 2. VORTEX2 during the time of VORTEX2 data collection, it did not spawn a tornado despite producing a well-de- The requisite visual, kinematic, and thermo- fined wall cloud. dynamic data to examine the aforementioned wall cloud formation mechanisms was collected during 3. DATA ANALYSIS METHODS the Verification of the Origins of Rotation in Torna- does Experiment II (VORTEX2). VORTEX2 was The wind field in and around the wall clouds a large multi-agency field project designed to col- was generated from dual-Doppler synthesis of radial 2 velocities collected by the mobile X-band Doppler cup anemometer. All data, including GPS derived On Wheels (DOW) 6 and 7 radars operated by the latitude and longitude, were collected at one-second Center for Severe Weather Research (CSWR; Wur- intervals. Data collected within the window of five man 2001). After the data were edited and navigat- minutes before and after the dual-Doppler analysis ed, it was objectively interpolated to a Cartesian grid time were used in the analyses. It was assumed using a two-pass Barnes scheme. The horizontal that storm evolution was not significant during this and vertical grid spacing was set to 100 m over a ten-minute time period. All mobile mesonet data 15 km x 15 km horizontal domain. Vertical veloc- were space-time adjusted to account for storm mo- ities were calculated by upward integration of the tion. mass continuity equation. Dual-Doppler volumes The visual extent and evolution of the wall were collected every two minutes. Examples of du- clouds was determined by photogrammetry analysis al-Doppler wind syntheses from VORTEX2 data and of still images captured by two photo teams. Pho- further details of the dual-Doppler technique can be togrammetry is the process of superimposing azi- found in Markowski et al. (2012a, b), Atkins et al. muth and elevation angle grids on photographs by (2012), and Kosiba et al. (2013). computing or determining the precise azimuths of The thermodynamic properties of low-level landmarks in the horizon relative to the photogra- parcels entering the wall cloud, was based on mo- pher. Once the landmark locations are known, the bile mesonet (Waugh and Fredrickson 2010) obser- effective focal length and tilt angle of the photograph vations. The mobile mesonets carried roof-mounted can be computed. Spherical trigonometry is then instrumentation that included temperature, relative used to create the azimuth-elevation grid. Once the humidity, and pressure sensors along with a three- photo has been gridded, it can then be combined 5 June, 2009 Photo Time: 2200:24 UTC CAMA (a) Mesonet estimated 3° wall cloud base: 530 m ±50 m 560 m Wall Cloud 400 m 1° 274° 276° 278° 280° 281.4° 282° 284° 286° 288° 5 June, 2009 Photo Time: 2200:33 UTC CAMB (b) 3° Wall Cloud 1° 336° 338° 340° 342° 344° 346° FIG 3. Photographs of the wall cloud taken at (a) 2200:24 UTC from the CAMA and (b) 2200:33 UTC from the CAMB locations, respectively on 5 June 2009. Photogrammetric and mobile mesonet estimates of wall cloud base height are shown in black and purple, respectively. Wall cloud boundary is shown in red. 3 5 June, 2009 2200 - 2202 UTC z = 600 m Reflectivity (dBZ) W (ms-1) horizontal winds p’ (mb) -1 285° from CAMA location of minimum OW 281.4° 276° -3 approximate wall cloud location 344° from CAMB 344° from CAMB -1 -1 0 mb 1 337° 337° 1 4 km (a) 30 ms -1 (b) 0 5 10 15 20 25 dBZ 35 40 45 50 55 -20 -15 -10 -5 0 ms-1 10 15 20 FIG 4. Same as Fig. 2 except for the time period 2200:00 – 2202:00 UTC. with the dual-Doppler wind field and radar reflectivity Consistent with Fig. 1, the wall cloud was embed- observations. More details on photogrammetry can ded in precipitation that was wrapping around and be found in Wakimoto et al. (2011) and Atkins et al. embedded in the low-level mesocyclone (Fig. 1a). (2012). Video (not shown) revealed that the wall cloud con- tained significant rotation. The strongest rotation 4. GOSHEN COUNTY TORNADIC WALL CLOUD in the dual-Doppler data was located by computing and locating the minimum Okubo-Weiss number 4.1 Visual and Dual-Doppler Observations (Okubo 1970; Weiss 1991; Markowski et al.
Details
-
File Typepdf
-
Upload Time-
-
Content LanguagesEnglish
-
Upload UserAnonymous/Not logged-in
-
File Pages12 Page
-
File Size-