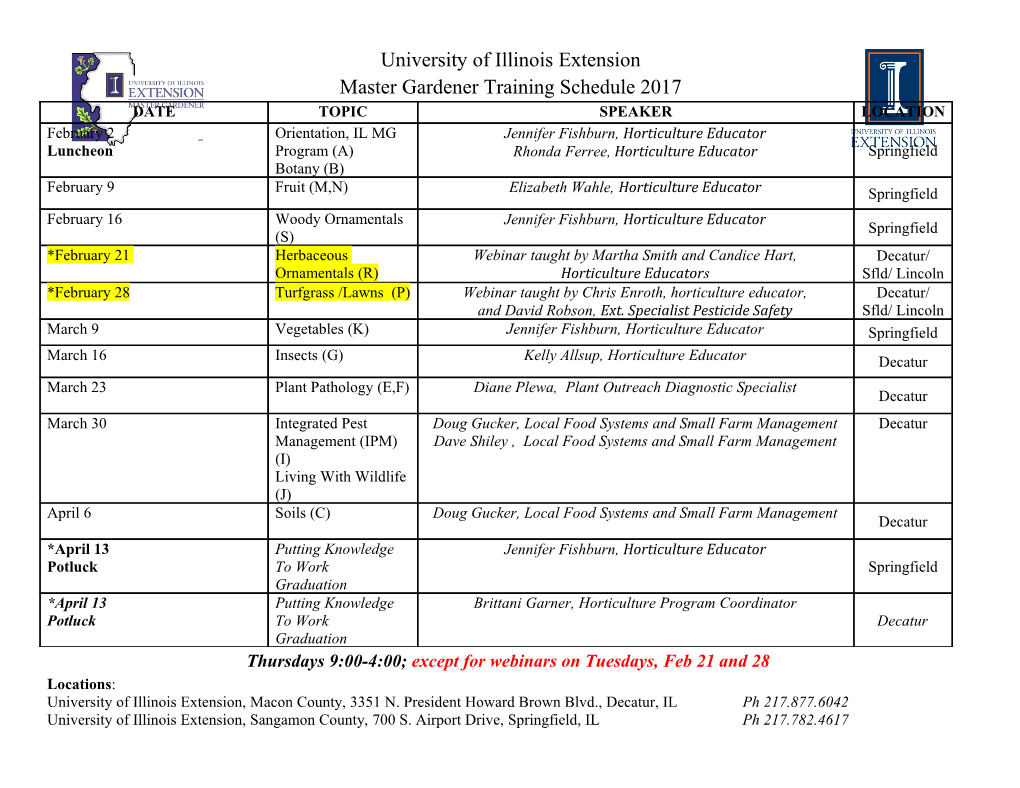
Downloaded from http://cshperspectives.cshlp.org/ on September 25, 2021 - Published by Cold Spring Harbor Laboratory Press Sources of DNA Double-Strand Breaks and Models of Recombinational DNA Repair Anuja Mehta and James E. Haber Rosenstiel Basic Medical Sciences Research Center, MS029 Rosenstiel Center, Brandeis University, Waltham, Massachusetts 02454-9110 Correspondence: [email protected] DNA is subject to many endogenous and exogenous insults that impair DNA replication and proper chromosome segregation. DNA double-strand breaks (DSBs) are one of the most toxic of these lesions and must be repaired to preserve chromosomal integrity. Eukaryotes are equipped with several different, but related, repair mechanisms involving homologous re- combination, including single-strand annealing, gene conversion, and break-induced rep- lication. In this review, we highlight the chief sources of DSBs and crucial requirements for each of these repair processes, as well as the methods to identify and study intermediate steps in DSB repair by homologous recombination. EXOGENOUS AND ENDOGENOUS Some well-known exogenous DNA damag- SOURCES OF DNA DOUBLE-STRAND ing agents (clastogens) are anticancer chemo- BREAKS therapeutic drugs and ionizing radiation (IR). Chemotherapeutic drugs include DNA-alkyl- NA damage can occur as a result of en- ating agents such as methyl methanosulfo- Ddogenous metabolic reactions and replica- nate and temozolomide, cross-linking agents tion stress or from exogenous sources like radi- such as mitomycin C and cisplatin, and radio- ation and chemotherapeutics. Damage comes mimetic compounds such as bleomycin or in several different varieties: base lesions, intra- phleomycin (Chen and Stubbe 2005; Wyrobek and interstrand cross-links, DNA-protein cross- et al. 2005). Another class are topoisomerase links, and both single- and double-strand inhibitors such as camptothecin and etoposide, breaks (DSBs) (Lindahl 1993). Some types of which induce the formation of single-strand damage, such as oxidative damage to DNA bas- breaks (SSBs) and DSBs, respectively, by trap- es, arise, and are repaired, as often as 105 lesions ping covalently linked topoisomerase-DNA per cell each day (Hoeijmakers 2009). Much less cleavage complexes (Koster et al. 2007). Other frequent are DNA DSBs, in which the phosphate drugs, such as hydroxyurea and aphidicolin, backbones of the two complementary DNA impair the progression of replication by deplet- strands are broken simultaneously, and these ing deoxyribonucleotide pools or inhibiting are one of the most cytotoxic forms of lesion. DNA polymerase. Editors: Stephen Kowalczykowski, Neil Hunter, and Wolf-Dietrich Heyer Additional Perspectives on DNA Recombination available at www.cshperspectives.org Copyright # 2014 Cold Spring Harbor Laboratory Press; all rights reserved; doi: 10.1101/cshperspect.a016428 Cite this article as Cold Spring Harb Perspect Biol 2014;6:a016428 1 Downloaded from http://cshperspectives.cshlp.org/ on September 25, 2021 - Published by Cold Spring Harbor Laboratory Press A. Mehta and J.E. Haber Ionizing radiation leads to extensive base chik and Knudson 2003). In vertebrate cells damage and, additionally, creates DNA SSBs such as chicken DT40, depleted for yet another by producing radiolysis radicals that attack key recombination protein, Rad51, the estimat- the sugar-phosphate backbone (Ward 1994; ed rate of breakage is of the same magnitude Thompson 2012). Frequently, at high doses of (Sonoda et al. 2001). irradiation, two such nicks are present in com- An alternate way to count DSBs in a cell is to plementary DNA strands within one helical monitor the formation of damage-induced foci, turn leading to DSBs (Milligan et al. 1995). either by indirect immunofluorescent stain- There are about 10 SSBs for each DSB created ing or the use of fluorescent proteins fused to by IR (Ma et al. 2012). IR breakage frequently proteins that are recruited to the sites of DNA leaves “dirty ends,” consisting of phosphoglyco- damage as part of the DNA damage response. lates and terminal nucleotides, that cannot be In vertebrate cells, phosphorylation of the mi- ligated to “clean” ends consisting of a 50 phos- nor histone H2Avariant, H2AX, to produce so- phate and 30-OH group, such as those created by called g-H2AX, is often used as an indicator of endonucleases (Weinfeld and Soderlind 1991). the incidence of DSBs; however, it is now be- Even in the absence of exogenously inflicted coming evident that g-H2AX can be associated stress during an unperturbed cell cycle, DNA with DNA damage other than DSBs (Soutoglou is vulnerable to suffer damage during replica- and Misteli 2008; Lo¨brich et al. 2010; Valdigle- tion, which, if unrepaired, can promote geno- sias et al. 2013) and thus may overestimate their mic instability. There are numerous natural incidence. Binding of other key DNA repair pro- impediments that lead to pausing or blocking teins,such as 53BP1, also serves as asurrogate for of a replication fork, such as unusual DNA and monitoring DSBs, as do the appearance of RPA chromatin structures or collisions with tran- (replication protein A) and Rad51 foci (Haaf scription machinery (Prado and Aguilera 2005; et al. 1995; Raderschall et al. 1999; Noon and Aguilera and Gaillard 2014) or DNA-binding Goodarzi 2011). In budding yeast, the most fre- proteins (Mirkin and Mirkin 2007; Merrikh quently used live-cell marker of DSB damage is et al. 2012). The types of damage produced the recruitment of Rad52-YFP (or other colors) by normal cellular processes are very similar to into damage-induced foci. The fact that even those caused by some environmental agents (De multiple DSBs result in a single Rad52 focus Bont and van Larebeke 2004). has been interpreted as evidence that DSBs ag- One way to estimate the frequency of spon- gregate into a repair center (Lisby et al. 2003); taneous DSBs is to count them in cells in which however, recent studies have suggested that DSB repair is prevented. In budding yeast, one Rad52-fluorescent protein fusion proteins have can examine the fate of a single G1 cell lacking a remarkable ability to aggregate, so that they the RAD52 gene that is required for DSB repair mark strongly only one of several independent by homologous recombination (HR). Approx- DSBs (M Brown, I Fitzgerald, B Glick, and D imately one cell in eight gives rise to a pair of Bishop, unpubl.; C-S Lee and J Haber, unpubl.). daughter cells, one of which is inviable (J Haber, The fact that the majority of spontaneous unpubl., cited in Coı¨c et al. 2008). This finding DSBs appear in the context of DNA replication implies that there is a DSB that arises during (Syeda et al. 2014) could suggest that DNA may DNA replication that would normally be re- be nicked before the passage of the replication paired by sister chromatid recombination in a fork, thus creating a broken chromatid, and, recombination-proficient cell. Given a genome with the arrival of another replication fork in size of 1.2 Â 107 bp, this result, hence, sug- the opposite direction, an intact sister chroma- gests that there is about one spontaneous DSB tid. However, such breaks can also arise by the per 108 bp. Another study estimates that, in nor- processing of stalled replication forks. Stalled mal human cells, 1% of single-strand lesions forks have been studied by impairing replica- are converted to 50 DSBs per cell per cell cy- tion, for example, by adding hydroxyurea or cle, that is, about one DSB per 108 bp (Vilen- introducing replication fork-blocking sequenc- 2 Cite this article as Cold Spring Harb Perspect Biol 2014;6:a016428 Downloaded from http://cshperspectives.cshlp.org/ on September 25, 2021 - Published by Cold Spring Harbor Laboratory Press Sources of DSBs and Their Repair by Recombination es. Stalled forks can regress by the unwinding and Aguilera 2003; Kim and Jinks-Robertson and annealing of the newly synthesized strands 2009; Lin et al. 2010). R-loops are mainly to form a “chicken foot” structure, which is formed by defects in RNA metabolism, but known as a Holliday junction (Fig. 1). These also seem to arise naturally in wild-type cells Holliday junctions (HJs) can be cleaved by (Helmrich et al. 2011; Wahba et al. 2011). It structure-selective nucleases or HJ resolvases, was recently reported that formation of these such as Mus81-Mms4 or Yen1, to create a bro- RNA:DNA hybrids, in certain yeast transcrip- ken end and intact chromatid (Wyatt and West tion repression and RNA degradation mutants, 2014). The broken end can then be processed requires the involvement of the HR machinery, by 50 to 30 resection (Symington 2014) and, after including Rad51 and Rad52 (Wahba et al. binding Rad51, engage in recombination-de- 2013). Several high-throughput screens in bud- pendent replication restart, that is, break-in- ding yeast and mammalian cells have identified duced replication (BIR) (Hanada et al. 2007; multiple RNA biogenesis factors whose deple- Petermann and Helleday 2010). tion leads to R-loops-mediated DSB formation A growing body of evidence implicates tran- and the activation of the DNA damage response scription as one of the leading causes of DSBs (Li and Manley 2005; Paulsen et al. 2009; Stir- and associated genome instability. Apart from ling et al. 2012; Gavalda et al. 2013). The evo- hindering replication fork progression (Prado lutionarily conserved RNase H (in prokaryotes) and Aguilera 2005), R-loops, three-strand nu- or RNase H1 (in eukaryotes), which specifically cleic acid structures formed by an RNA:DNA degrades the RNA portion of hybrids, plays a hybrid, plus a displaced single-stranded DNA major role in suppression and removal of such (ssDNA) identical to the RNA molecule, have structures and, hence, helps maintain genome been linked to mutagenesis, recombination, stability (Lin et al. 2010; Helmrich et al. 2011; and chromosome rearrangements (Huertas Wahba et al. 2011). Thus, a complex network A B C TT^ TT^ TT^ Fork stalling Fork regression and Holliday junction cleavage Holliday junction formation D E F TT ^ TT ^ TT ^ Fork collapse by Holliday Strand invasion Replication restart junction cleavage Figure 1.
Details
-
File Typepdf
-
Upload Time-
-
Content LanguagesEnglish
-
Upload UserAnonymous/Not logged-in
-
File Pages19 Page
-
File Size-