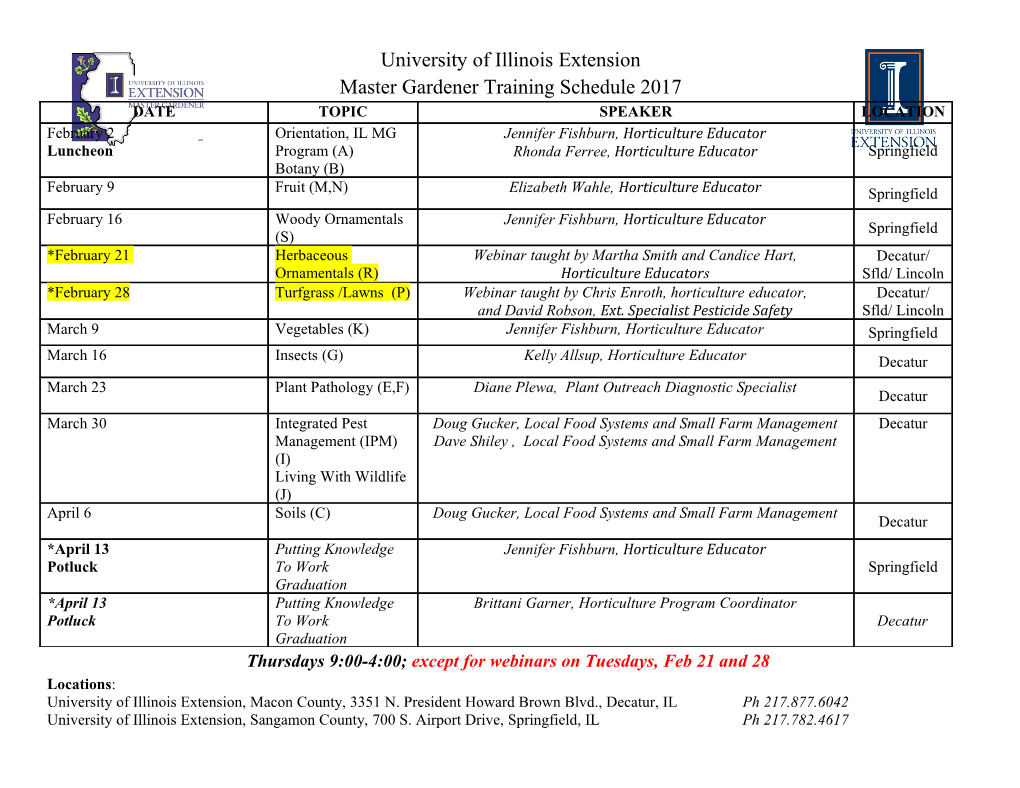
Chapter 7 Nucleosynthesis in Supernovae The explosion of a core-collapse supernova leads to ejection of the star's mantle, and thus to substantial enrichment of the interstellar medium with the major burning products of hydrostatic equilibirium: 4He, 12C, 16O, 20Ne, etc. As was described in the first lecture of this course, these are among the most plentiful elements in nature. However neither this mechanism nor any other process we have discussed so far describes how many other ele- ments found in nature are synthesized. In this chapter several mechanisms associated with the explosive conditions of a supernova - explosive nucleosynthesis, the s- and r-processes, and the neutrino process - will be described. 6.1 Explosive nucleosynthesis and the iron peak The creation of elements by the explosion itself - e.g., the high temperatures associated with passage of the shock wave - is called explosive nucleosynthesis. The properties of this process are tied to those of the explosion, which we have seen are still poorly understood. But the observation that the kinetic energy of a supernova explosion is typically in the range of (1-4) 1051 ergs, provides an important constraint. · Estimates of this synthesis depend on a number of issues: Description of the presupernova model. The conventional approach is to evolve a star of given• initial composition (e.g., metal content) through the various burning stages. The result is influenced by the assumed initial metallicity, the nuclear cross sections adopted, and the phyical mechanisms modeled, such as mass loss and convection. The galactic model. Presumably the abundances we see today result from integrating over a• large number of events. Thus one needs to know the characteristics of typical supernovae, e.g., the distribution of Type II supernovae over possible masses. And one has to take ac- count of evolutionary effects: are the number of large stars in the early history of our galaxy similar to today? How does the supernova rate evolve? Was there an early \bright phase" in our galaxy's evolution? Do overall changes in our galaxy, such as its metallicity, have an effect on supernova characteristics? Reaction rates. Our information about exotic nuclear reactions - reactions involving ex- cited• states, unstable nuclei that have not been studied in the laboratory, or simply reactions that have not been measured - often is too limited. Thus there is always some change being made due to new information from laboratory measurements. For a mass point well away from the neutrinosphere - perhaps 30,000 kilometers from the center of the star - it is a reasonable approximation to assume the density and temperature of the matter are changed little during the explosion until the shock wave arrives at that point. There is an approximate expression for the time of shock arrival s Mr MNS t0 0:7r9 − s (1) ∼ E51 1 9 51 where r9 is the radius of point in 10 km, E51 is the energy of the explosion in 10 ergs, MNS 1:4 solar masses is the mass of the newly formed neutron star, and Mr is the Lagrangian∼ mass coordinate of the shell in question. Under the asumption that the part of the supernova behind the shock front is approximately isothermal and that the energy is contained in the radiation field, one might expect the peak temperature Tp produced by the shock wave to be 4πr3 π2k4T 4 E = o (2) 3 × 15(¯hc)3 or equivalently 4πar3 E = T 4 o (3) p · 3 15 3 4 where a is the radiation constant (a = 7.56 10− ergs/cm K and is related to the Stefan Boltzmann constant by σ = ac=4). The equation× above says that the energy density of radition (aT 4) times the volume gives the explosion energy. Numerically a relation is obtained that is quite compatible with this simple picture 9 1=4 3=4 T 2:4 10 E r− (4) p ∼ × 51 9 For a 20 solar mass star (from Woosley et al.) one gets the following results Shell Radius T Tp Density Mass tshock (cm)(K)(K)(g=cm3)(solar)(s) hydrogen 1:00E11 8:00E6 7:59E7 1:0E 2 6:26 154:3 helium 1:51E10 1:02E8 3:13E8 5:57E−1 4:79 19:46 (5) carbon 3:44E9 3:68E8 9:50E8 4:93E3 3:54 3:52 neon 1:78E9 7:56E8 1:56E9 3:28E4 2:73 1:44 oxygen 4:33E8 2:11E9 4:50E9 8:41E5 1:79 0:19 silicon 2:89E8 2:88E9 6:09E9 2:08E6 1:65 0:10 The numerical values are for the centers of each shell except for carbon, where the values are for the inner part of that shell. The difference between Tpeak and T shows the elevated temperature that results from shock wave passage. Note that Tp reaches several in units of T9 in the inner (silicon, oxygen, neon) shells. The resulting soup of photons, αs, and nucleons of several hundred keV can clearly process ma- terial in these shells. In the silicon shell significant production of iron-peak elements results: 56;57Fe, 59Co, 58;60;61;62Ni, 63;65Cu, 64;66Zn. Other important productions includes 44Ca, 48Ti, etc. The pattern is quite similar in the oxygen shell. The somewhat lower Tp characteriz- ing the neon shell shifts the synthesis to somewhat lighter nuclei, e.g., 28Si, 32S, 36Ar, 41K, 40;42Ca, etc. Note many of these specifies can be formed by α capture reactions that are facilitated by the higher temperatures. Outside the neon shell, very little explosive synthesis occurs. 2 Solar Abundances 12 10 8 ] 6 6 4 Abundance [Si=10 10 log 2 0 -2 0 20 40 60 80 100 120 140 160 180 200 Mass Number Fig T h e ab u n d an ces of is otop es in th e s olar s ys tem as a f u n ction of atom ic m as s Figure[3,4]. 1: The Abundance abundances of are the normalized elements, so plotted that the as total a function abundance of the of silicon mass numberis 106. A. nuclear reaction played in the synthesis of the elements. In 1957, Burbidge, It is quiteBurbidge, possible Fowler that individual & Hoyle [1] regions and Cameron of the ejected [2] wove mantle these threa mayds remain into a more co- or less intact onhesive ejection, theory thereby of nucleosynthesis, allowing observers demonstrating to study how the the processes solar isotopic that occurred abun- in each shell individually.dances (displayed Around in 300-year-old Fig. 1) bore the supernova fingerprints remnant of their Cas astrophysical A regions have origins. been found Today, investigations refine our answers to these same two questions, how are that are strongly overabundant in elements such as S, Ar, and Ca. Another exciting pos- the elements that make up our universe formed, and how do these nuclear sibility { discussed over the past five years { is to use the composition of individual stellar transformations, and the energy they release, affect their astrophysical hosts. grains to determine not only the conditions under which specific isotopes are synthesized, but alsoIn the this specific article, chemistry we will concentrate connected with on summarizing the ejection the and two cooling basic of numerical the material from which thesemethods grains used condensed. in nucleosynthesis studies, the tracking of nuclear transmuta- tions via rate equations and via equilibria. We will also briefly discuss work 6.2 Abundanceswhich seeks above to meld the iron these peak methods and neutron together capture in order to overcome the limita- Figure 1tions shows of each. the abundance To properly pattern orient readers found in unfamiliar our solar with system. nuclear We astrophysics see abundant light nuclei, especiallyand to briefly the H, describe4He, and the light differing elements. physical An conditions abundance whi peakch influencenear the iron the isotopes is seen.optimal Then there choice are of lower abundance abundances evolution for heavier method, isotopes, we begin but with alsoa interesting brief intro- structure: mass peaksduction are seen to the around background A 130 astrophysics and 190. ( The2), before low-mass discussing structure the (at form and that below iron) ∼ ∼ reflects athe general rate equations tendancy take for Coulomb ( 3). In 4 barriers we will§ to discuss inhibit the synthesis difficulties of inherentincreasingly in heavier § § nuclei, withsolving the these irongroup rate equations. being exceptional5 describes because the equations it is favored of nuclear by its strongequilibria binding. as well as the limitations of§ their use. Finally in 6 we will discuss hybrid § schemes which seek to use local equilibria to simplify the rate equations. 3 2 Figure 2: This more detailed view of the heavy-mass abundance distribution shows sharp peaks near A=208 and 138, associated with the s-process, and broader peaks at somewhat lower mass, associated with the r-process. From Rauscher and Thielemann. A blowup of the pattern of heavy elements shows a clear structure associated with the closed neutron shells in nuclear physics: the stablest configurations are at the closed shells N =50, 82, and 126. There is a splitting of the abundance peaks, suggesting that perhaps there are two processes of interest. One can also see that the integrated abundance above the iron peak is not large, comparable to about 3% of the iron peak. Thus the processes responsible can be reasonably rare. This synthesis is associated with the neutron-capture reaction (n; γ). There are sources of neutrons in stellar interiors, and neutron capture cross sections on heavy nuclei can be quite large. We will also see that the observed shell structure is natural for such a process.
Details
-
File Typepdf
-
Upload Time-
-
Content LanguagesEnglish
-
Upload UserAnonymous/Not logged-in
-
File Pages23 Page
-
File Size-