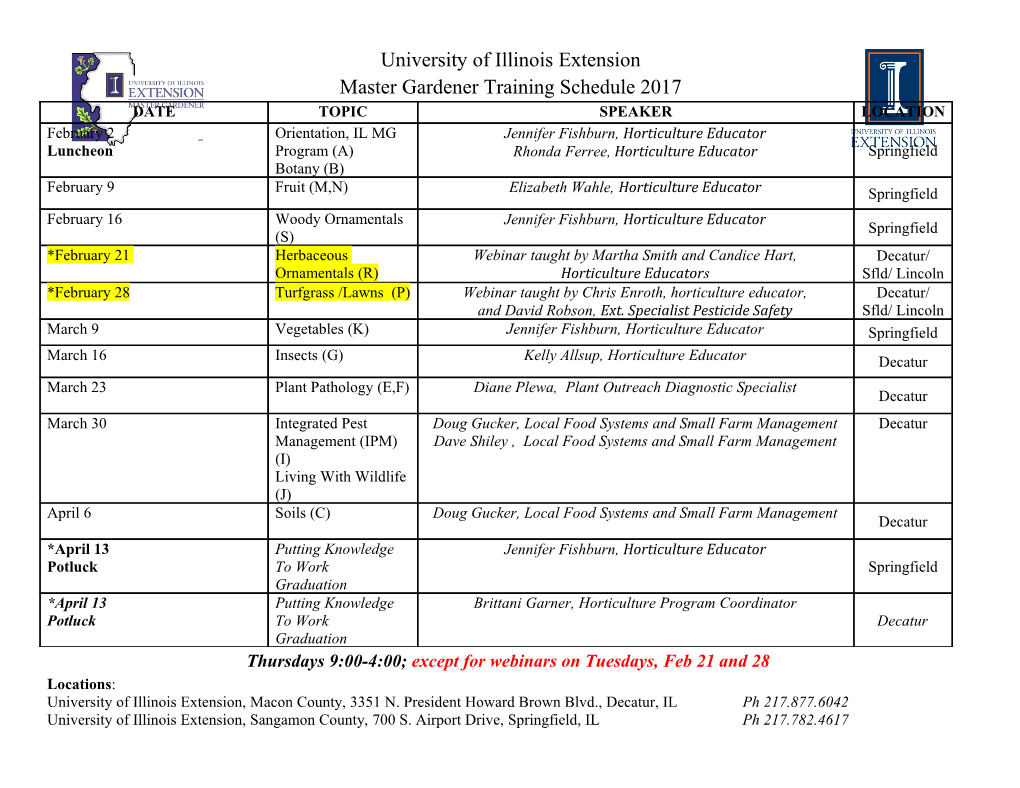
Meteoritics & Planetary Science 1–28 (2010) 1 doi: 10.1111/j.1945-5100.2010.01153.x 2 3 4 5 Almahata Sitta (=asteroid 2008 TC3) and the search for the ureilite parent body 6 7 Peter JENNISKENS1*,Je´ re´ mie VAUBAILLON2, Richard P. BINZEL3, Francesca E. DeMEO3,4, 8 5 5 6 7 9 David NESVORNY , William F. BOTTKE , Alan FITZSIMMONS , Takahiro HIROI , 1 1 8 9 10 Franck MARCHIS , Janice L. BISHOP , Pierre VERNAZZA , Michael E. ZOLENSKY , 9 10 11 12 11 Jason S. HERRIN , Kees C. WELTEN , Matthias M. M. MEIER , and Muawia H. SHADDAD 12 13 1SETI Institute, Carl Sagan Center, 189 Bernardo Ave, Mountain View, California 94043, USA 2 14 Observatoire de Paris, I.M.C.C.E., 77 Av. Denfert Rochereau, Bat. A., FR-75014 Paris, France 3 15 Department of Earth, Atmospheric, and Planetary Sciences, Massachusetts Institute of Technology, 16 77 Massachusetts Ave., Cambridge, Massachusetts 02139–4307, USA 4Observatoire de Paris, L.E.S.I.A., 5 Place Jules Janssen, FR-92195 Meudon, France 17 5Department of Space Studies, SWRI, 1050 Walnut St., Suite 400, Boulder, Colorado 80302, USA 18 6Astrophysics Research Centre, School of Mathematics and Physics, Queen’s University Belfast, 19 Belfast BT7 1NN, UK 20 7Department of Geological Sciences, Brown University, Providence, Rhode Island 02912, USA 21 8ESA, ESTEC, Keplerlaan 1, NL-2200 AG Noordwijk, The Netherlands 22 9NASA Johnson Space Center, 2101 NASA Parkway, Houston, Texas 77058, USA 23 10Space Sciences Laboratory, University of California, Berkeley, California 94720–7450, USA 24 11Department of Earth Sciences, E.T.H. Zurich, CH-8092 Zurich, Switzerland 12 25 1 Department of Physics and Astronomy, University of Khartoum, P.O. Box 321, Khartoum 11115, Sudan * 26 Corresponding author. E-mail: [email protected] 27 (Received 28 November 2009; revision accepted 25 October 2010) 28 29 Abstract–This article explores what the recovery of 2008 TC3 in the form of the Almahata 30 Sitta meteorites may tell us about the source region of ureilites in the main asteroid 31 belt. An investigation is made into what is known about asteroids with roughly the same 32 spectroscopic signature as 2008 TC . A population of low-inclination near-Earth asteroids is 33 3 identified with spectra similar to 2008 TC3. Five asteroid families in the Main Belt, as well 34 as a population of ungrouped asteroids scattered in the inner and central belts, are 35 identified as possible source regions for this near-Earth population and 2008 TC3. Three of 36 the families are ruled out on dynamical and spectroscopic grounds. New near-infrared 37 spectra of 142 Polana and 1726 Hoffmeister, lead objects in the two other families, also 38 show a poor match to Almahata Sitta. Thus, there are no Main Belt spectral analogs to 39 Almahata Sitta currently known. Space weathering effects on ureilitic materials have not 40 been investigated, so that it is unclear how the spectrum of the Main Belt progenitor may 41 look different from the spectra of 2008 TC3 and the Almahata Sitta meteorites. Dynamical 42 arguments are discussed, as well as ureilite petrogenesis and parent body evolution models, 43 but these considerations do not conclusively point to a source region either, other than that 44 2008 TC probably originated in the inner asteroid belt. 45 3 46 47 48 INTRODUCTION shape of the 550–1000 nm reflectance spectrum 49 measured prior to impact and the 300–2500 nm 50 On October 7, 2008, a small 3–4 m sized asteroid reflectance spectra of the earliest recovered meteorites 51 called 2008 TC3 impacted the Earth’s atmosphere over (Jenniskens et al. 2009). 52 2 the Nubian Desert of northern Sudan (Kowalski 2008). Meteorites collectively called Almahata Sitta were 53 The asteroid was classified as belonging to taxonomic recovered in the following months, scattered along the 54 class F, meaning ‘‘flat’’ (Tholen 1984), based on the flat approach path of the asteroid. Most of these fragments 1 Ó The Meteoritical Society, 2010. MAPS1153-1267 Dispatch: 13.11.10 Journal: MAPS CE: Vijay Journal Name Manuscript No. B Author Received: No. of pages: 28 PE: Karpagavalli 2 P. Jenniskens et al. 1 were ureilites of a wide range of types, some hampered by the fact that the larger Main Belt asteroids 2 anomalously porous compared to known ureilites. Thus, can be covered in different degrees by a layer of light- 3 Almahata Sitta was classified as an anomalous polymict scattering dust and regolith, scattering blue light more 4 ureilite (Zolensky et al. 2010). This provided the first than red, and thus causing a blue-tilted slope in 5 firm link between a meteorite type and an asteroid reflectance spectra (Gaffey et al. 1993; Burbine et al. 6 taxonomic class (Jenniskens et al. 2009). 2002). 2008 TC3, however, had a reflection spectrum 7 The result was surprising, because ureilites were very similar to that of the freshly fallen meteorites 8 traditionally linked to S-class (S for ‘‘stony’’) asteroids (Jenniskens et al. 2009; Hiroi et al. 2010), possibly 9 of subtype III (Gaffey et al. 1993; Sandford 1993; because this small tumbling asteroid rotated too fast to 10 Burbine et al. 2002), mainly based on ordinary hold on to surface regolith (Scheirich et al. 2010). 11 chondrite like visible slopes in ureilite reflectance spectra In addition, asteroid surfaces are irradiated by 12 now understood to be the result of terrestrial cosmic rays and solar wind, a process called space 13 weathering. For a comparison of Almahata Sitta weathering, and are bombarded by micrometeorites, a 14 reflectance spectra with S-class asteroid spectra, see process called impact gardening. Space weathering 15 Hiroi et al. (2010). Before this link was made, F- and B- causes nanophase iron to separate out of minerals, 16 class asteroids (B for ‘‘blue-sloped’’) did not seem to changing the surface reflectivity over time (lowering the 17 have meteorite analogs (Britt et al. 1992), or were linked asteroid’s geometric albedo for high albedo asteroids, or 18 to partially altered carbonaceous meteorites that are raising the albedo for dark asteroids), and typically red- 19 now thought to have better spectral analogs among K- tilting the reflectance slope at optical wavelengths and 20 complex asteroids, instead (Clark et al. 2009). somewhat less so at near-infrared (IR) wavelengths 21 In this article, we explore this link further and (Hiroi et al. 1999; Sasaki et al. 2002; Strazzulla et al. 22 attempt to use the first recovery of samples from a 2004; Brunetto et al. 2006; Marchi et al. 2006; Willman 23 known asteroid to search for the source region of et al. 2010). This process was first documented for the 24 ureilites in the asteroid belt. At first, this may seem an Moon (e.g., Pieters et al. 1993, 2000; Noble and Pieters 25 easy task, because the rarity of F-class asteroids (only 2003) and then observed for Mercury (Hapke 2001) and 26 4%, 92 of 2000 classified asteroids) and ureilites (only asteroids (e.g., Clark et al. 2002; Brunetto et al. 2006). 27 0.6% of meteorite falls, 6 of 987) suggests that the Because the 2008 TC3 asteroid spectrum was so similar 28 source region in the asteroid belt is a spectral anomaly. to that of the recovered meteorites, space weathering 29 Also, ureilites appear to have an unusual petrogenesis must not have affected the surface much in this 30 and protoplanet evolution that may provide additional wavelength range. 2008 TC3 broke off from a larger 31 insight into the source region. asteroid only 19.5 ± 2.5 Ma, based on cosmic ray 32 The obvious approach would be to compare the exposure (CRE) time (Welten et al. 2010). By contrast, 33 spectrum of 2008 TC3 to that of other asteroids and the Main Belt progenitor of 2008 TC3 was exposed for 34 select those that are most similar. In practice, however, a much more significant period of time before the 35 meteorite reflectance spectra are not necessarily the impact occurred that broke off 2008 TC3. Sadly, 36 same as asteroid reflectance spectra, and near-Earth nothing is known about how space weathering changes 37 asteroids (NEA) surfaces can differ from those of their the reflectance properties of ureilites. 38 parent asteroids in the Main Belt. For example, Q-class The reflectance spectra of asteroids can also be 39 asteroids are found in the Main Belt only in very young changed by thermal metamorphism (e.g., Clark et al. 40 <1 Myr families (Mothe´ -Diniz and Nesvorny 2008), 1992; Hiroi et al. 1993; Ohtsuka et al. 2009), although 41 but are common among NEA (e.g., McFadden et al. this is unlikely to be a concern in this case because 2008 42 1984; Fevig and Fink 2007), where they appear to be TC3 did not come close to the Sun in its recent orbital 43 examples of S-class asteroids that have had their evolution (see below). A more realistic, common 44 regolith disturbed and possibly overturned by concern is terrestrial weathering of the meteorites. 45 gravitational perturbations from close encounters with Terrestrial weathering rusts metallic iron into limonite 46 3 Earth (Binzel et al. 2010a, 2010b). In addition, many (iron oxides and hydroxides) with strong near-UV and 47 aspects of ureilite petrogenesis and protoplanet 900 nm absorption bands (Cloutis et al. 2010; Hiroi 48 evolution are too controversial and model-dependent to et al. 2010). However, Almahata Sitta is the first 49 provide firm constraints. polymict ureilite not severely affected by terrestrial 50 weathering (Zolensky et al. 2010). 51 Linking Meteorite Types to Asteroid Taxonomic Classes Other aspects that complicate linking meteorites to 52 their parent bodies in the Main Belt include 53 Earlier attempts to identify parent bodies of inhomogeneity in the asteroid progenitors on a 54 particular meteorite groups in the Main Belt have been macroscopic scale.
Details
-
File Typepdf
-
Upload Time-
-
Content LanguagesEnglish
-
Upload UserAnonymous/Not logged-in
-
File Pages33 Page
-
File Size-