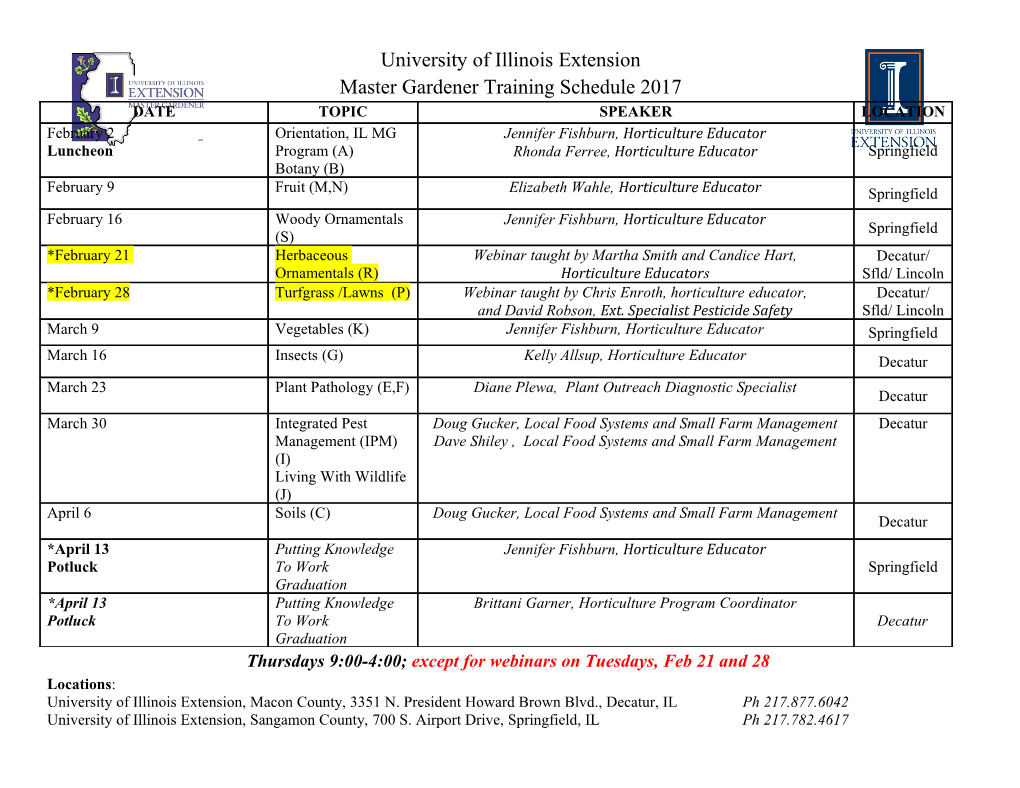
A new dawn: gravitational wave observations from Earth and in space Emanuele Berti, Johns Hopkins University Emera Astronomy Center Orono (ME), November 14 2019 Planetarium show credits: Nicolas Yunes School of Film, the Department of Physics and the Museum of the Rockies A brief history of black holes Black holes: the early story November 1915: ✓ Einstein presents the equations of general relativity to the Prussian Academy of Science 1916: ✓ Karl Schwarzschild discovers black holes while in the German army during World War 1. Schwarzschild dies of a disease developed on the Russian front Einstein does not believe in the physical reality of the Schwarzschild solution… 1930: ✓ 19-year old Subramanyan Chandrasekhar wins a Scholarship to study in Cambridge. On the boat to England, combining quantum mechanics and relativity, he discovers that massive white dwarfs must collapse gravitationally. Sir Arthur Eddington ridicules him: “stellar buffoonery” 1937: ✓ Chandra moves to Chicago 1983: ✓ Chandra receives the Nobel prize Black holes: the early story September 1939: ✓ World War 2 begins ✓ Oppenheimer & Snyder understand that collapse can lead to a black hole 1941: ✓ Oppenheimer stops working on relativity to lead the Manhattan Project 1939: ✓ John Wheeler & Niels Bohr study nuclear fission ✓ Wheeler’s brother dies in Italy; he joins the Manhattan project 1950s: ✓ Wheeler and his students (including Kip Thorne) return to the problem of gravitational collapse 1973: ✓ Misner-Thorne-Wheeler, “Gravitation” Black holes: the Golden Age (1963-1970s) Late 1960s and 1970s: ✓ “Golden age” of black hole physics ✓ Kip Thorne and students (including Saul Teukolsky) prove stability and understand the dynamics of black holes 1963: ✓ Roy Kerr from New Zealand discovers a mathematical solution describing rotating black holes ✓ Maarten Schmidt at Caltech discovers the first quasar, 3C273 at z=0.15 ✓ Must be compact and outshines the brightest galaxies! ✓ First supermassive black hole Singularity theorems, the Big Bang, and Hawking radiation ✓ Roger Penrose proves that gravitational collapse inevitably leads to singularities ✓ Stephen Hawking proves that the Universe must have been born out of a singularity… unless quantum mechanics comes into play ✓ Hawking radiation: connection between general relativity and quantum mechanics? Black holes: truth and beauty “In my entire scientific life, extending over forty-five years, the most shattering experience has been the realization that an exact solution of Einstein's equations of general relativity, discovered by the New Zealand mathematician, Roy Kerr, provides the absolutely exact representation of untold numbers of massive black holes that populate the universe. This shuddering before the beautiful, this incredible fact that a discovery motivated by a search after the beautiful in mathematics should find its exact replica in Nature, persuades me to say that beauty is that to which the human mind responds at its deepest and most profound.” (S. Chandrasekhar) How do black holes form? The life and death of stars Life: ✓ Gravity vs. pressure from nuclear burning ✓ Nuclear fusion produces heavy elements ✓ Iron is too stable, the star runs out of fuel Death: ✓ Gravity wins! Supernova ✓ Atoms heavier than iron formed in a supernova or neutron star merger ✓ Crab nebula (1054): seen by Chinese astronomers for 23 days during the day, and 2 years at night ✓ Crab left behind a neutron star The stellar graveyard (or: the life of stars after death) M<3Msun 3Msun<M< ~10Msun http://essayweb.net/astronomy/blackhole.shtml Visualizing gravity: curvature and “embedding diagrams” Thorne’s “parable of the ants” 6 intelligent ants live on a membrane and communicate by rolling balls at constant speed (as measured locally). 5 ants gather at the center: the membrane collapses and drags things inward. An “astronomer ant” keeps observing the balls, that arrive slower and slower. To her, the collapse seems to freeze. At t=15s, the astronomer ant stops receiving signals: the membrane is collapsing at the same speed as the balls move. However the collapsing star is not frozen! The five ants and the balls are all crushed into a central singularity. This is exactly what happens when a star collapses to a black hole. How can we see a black hole? How can we “see” a black hole? Sgr A* (Movie: Reinhard Genzel) 8 Andromeda (M31): 2.6 million lyrs away, 10 Msun black hole Radio Microwave Infrared Visible Ultraviolet X-Rays Gravity: Newton vs. Einstein Newton (1687): Einstein (1915): ✓ Action at a distance ✓ Gravity is spacetime curvature ✓ Describes effect of gravity, ✓ “Spacetime tells mass how to move, but does not explain it mass tells spacetime how to curve” Extreme light bending: EHT and M87’s light ring 9 55 million lyrs away, 6.5x10 Msun black hole Gravitational waves Gravitational waves as tidal forces 1 ton 2 m frot=1 kHz, h~(2.6 x 10-33 m)/r r > ~3x105 m: h < 9 x 10-39 M~1033 g, v=c, r~15 Mpc, Illustrations from Kip Thorne’s h~10-21 “Black holes and Time Warps: Einstein’s Outrageous Legacy” Interferometric detectors Interferometers ideal for the quadrupolar nature of gravitational waves: send laser beams in perpendicular directions and combine them on return to construct interference patterns. Building new ears Virgo GW150914: a new astronomy VIEWPOINT The First Sounds of Merging Black Holes Gravitational waves emitted by the merger of two black holes have been detected, setting the course for a new era of observational astrophysics. by Emanuele Berti⇤,† or decades, scientists have hoped they could “ lis- ten in” on violent astrophysical events by detecting their emission of gravitational waves. The waves, Fwhich can be described as oscillating distortions in the geometry of spacetime, were first predicted to exist by Einstein in 1916, but they have never been observed di- rectly. Now, in an extraordinary paper, scientists report that they have detected the waves at the Laser Interferometer Gravitational-wave Observatory (LIGO) [1]. From an analy- sis of the signal, researchers from LIGO in the US, and their collaborators from theVirgo interferometer in Italy, infer that the gravitational waves were produced by the inspiral and merger of two black holes (Fig. 1), each with a mass that is more than 25 times greater than that of our Sun. Their find- ing provides the first observational evidence that black hole binary systems can form and merge in the Universe. Gravitational waves are produced by moving masses, and Figure 1: Numerical simulations of the gravitational waves emitted like electromagnetic waves, they travel at the speed of light. by the inspiral and merger of two black holes. The colored Asthey travel, thewaves squash and stretch spacetime in the contours around each black hole represent the amplitude of the plane perpendicular to their direction of propagation (see gravitational radiation; the blue lines represent the orbits of the black holes and the green arrows represent their spins. (C. inset, Video 1). Detecting them, however, is exceptionally Henze/NASA Ames Research Center) hard because they induce very small distortions: even the strongest gravitational waves from astrophysical events are only expected to produce relative length variations of order 10− 21. phase, yielding no signal. A gravitational wave propagat- ing perpendicular to the detector plane disrupts this perfect “ Advanced” LIGO, as the recently upgraded version of destructive interference. During its first half-cycle, the wave the experiment is called, consists of two detectors, one in will lengthen one arm and shorten the other; during its sec- Hanford, Washington, and one in Livingston, Louisiana. ond half-cycle, these changes are reversed (see Video 1). Each detector is a Michelson interferometer, consisting of These length variations alter the phase difference between two 4-km-long optical cavities, or “ arms,” that are arranged the laser beams, allowing optical power—a signal—to reach in an L shape. The interferometer is designed so that, in the photodetector. With two such interferometers, LIGO can the absence of gravitational waves, laser beams traveling in rule out spurious signals (from, say, a local seismic wave) ◦ the two arms arrive at a photodetector exactly 180 out of that appear in one detector but not in the other. LIGO’s sensitivity is exceptional: it can detect length dif- ⇤Department of Physics and Astronomy, The University of Missis- ferences between the arms that are smaller than the size sippi, University, Mississippi 38677, USA of an atomic nucleus. The biggest challenge for LIGO is †CENTRA, Departamento de Física, Instituto Superior Técnico, detector noise, primarily from seismic waves, thermal mo- Universidade de Lisboa, Avenida Rovisco Pais 1, 1049 Lisboa, Por- tion, and photon shot noise. These disturbances can easily tugal mask the small signal expected from gravitational waves. physics.aps.org c 2016 American Physical Society 11 February 2016 Physics 9, 17 The sound of black holes 3 LIGO/Virgo: O1, O2 FIG. 1. Left: BNS range for each instrument during O2. The break at week 3 was for the 2016 end-of-year holidays. There was an additional break in the run at week• O1:23 to 9/12/2015make improvements-1/19/2016:to instrument sensiti3 vityBH. -TheBHMontana earthquake’ s impact on the LHO instrument sensitivity can be seen• at weekO2: 31.11/30/2016Virgo joined O2-8/25/2017:in week 34. Right: Amplitude7 BH-BHspectral + 1 densityNS-NSof the total strain noise of the Virgo, LHO and LLO detectors. The curves are representative of the best performance of each detector during O2. first-generation detector in 2011. The main modifications in- For the LIGO instruments this final calibration benefitted clude a new opticalhttps://gracedb.ligo.org/latest/design, heavier mirrors, and suspended from the use of post-run measurements and removal of instru- optical benches, includinghttps://www.gwphotodiodes in-openscience.org/detector_status/vacuum. Special mental lines. The calibration uncertainties are 3.8% in ampli- care was also taken to improve the decoupling of the instru- tudeand 2.1 degreesinphasefor LLO; 2.6% inamplitude and ment from environmental disturbances.
Details
-
File Typepdf
-
Upload Time-
-
Content LanguagesEnglish
-
Upload UserAnonymous/Not logged-in
-
File Pages49 Page
-
File Size-