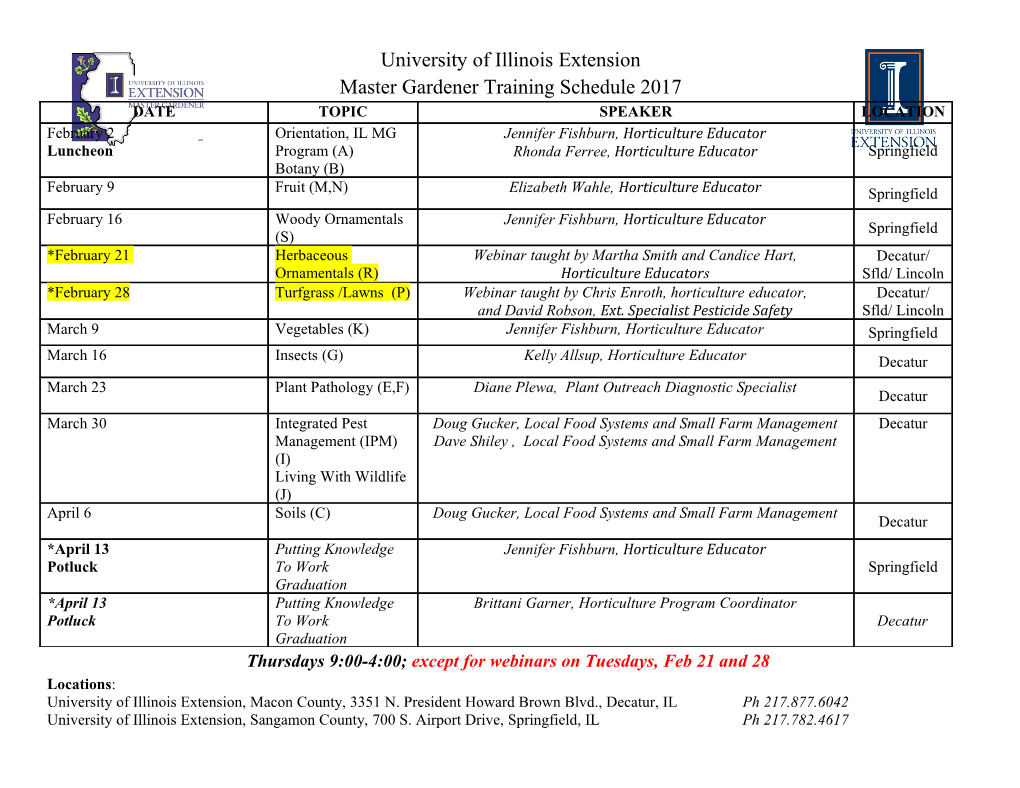
water Article Raindrop Energy Impact on the Distribution Characteristics of Splash Aggregates of Cultivated Dark Loessial Cores Yu Fu 1, Guanglu Li 1,2,*, Dong Wang 2, Tenghui Zheng 1 and Mingxi Yang 2 1 Institute of Soil and Water Conservation, Northwest A&F University, No. 26 Xinong Road, Yangling 712100, China 2 College of Resources and Environment, Northwest A&F University, No. 3 Taicheng Road, Yangling 712100, China * Correspondence: [email protected]; Tel.: +86-029-87082376 Received: 15 June 2019; Accepted: 18 July 2019; Published: 21 July 2019 Abstract: To determine the effect of different rainfall energy levels on the breakdown of soil aggregates, this study analyzed the soil splash erosion amounts and the distribution of particle sizes under six rainfall conditions (rainfall energy: 2.41 10 5–22.4 10 5 J m 2 s 1 and 1.29 10 4 J m 2 s 1) at five × − × − − − × − − − splash distances (from 0–10 cm to 40–50 cm). Cores of the size 10 20 cm of undisturbed cultivated × dark loessial soil were selected in tree replicates as the research subject. The results indicated that splashed aggregates were distributed mainly at splash distances of 0–20 cm, which accounted for 66%–90% of the total splash erosion amount. The splash erosion amount significantly decreased exponentially with increasing splash distance for the same rainfall energy (p < 0.01). The splash erosion amount significantly increased in the power function relationship with increasing rainfall energy at the same splash distance (p < 0.05). A model was obtained to predict the splash erosion amount for rainfall energy and splash distance. The fractal dimension (D) of the aggregates showed a downward opening parabolic relationship with raindrop energy. The maximal value of the rainfall energy was 1.286 10 4 J m 2 s 1, which broke the aggregates to the largest degree. Enrichment ratio × − − − (ER) values for fragments >2 mm were close to 0. A particle size of 0.25 mm was the critical particle level for splash erosion. Keywords: raindrop energy; soil aggregate; splash distance; fractal dimension; Loess Plateau 1. Introduction Soil erosion reduces land productivity and soil fertility, destroys farmland, exacerbates flood disasters, and results in soil environment deterioration, which affects land exploitation and the protection and utilization of soil and water resources [1,2]. Water erosion is the main type of soil erosion in the Loess Plateau. The aggregates caused by splash erosion are dispersed and broken, which is the initial stage of water erosion [1–4]. Raindrops fall from the air and impact the surface soil particles at a certain speed under the action of gravity. Some soil particles are separated from the soil and become loose particles, which is conducive to the formation and flow of surface runoff. At the same time, the process provides abundant loose particles for subsequent runoff transport [5,6]. Additionally, soil particle transport leads to reduced or blocked pores on the soil surface and reduced soil permeability due to soil crust formation [7–9]. At present, research on splash erosion can be divided into two methods: Natural rainfall and artificial simulated rainfall. The observation of natural rainfall requires a long timeframe, and many factors are difficult to control, which makes the collection of splash data difficult. Artificial simulated rainfall can make up for shortages of natural rainfall and improve the feasibility of testing and reliability Water 2019, 11, 1514; doi:10.3390/w11071514 www.mdpi.com/journal/water Water 2019, 11, 1514 2 of 11 of the data. Rainfall characteristics include raindrop diameter, rainfall intensity, raindrop kinetic energy, and so on. The effect of rainfall intensity on raindrop diameter and rainfall was studied by Yakubu et al. [10], who proposed that the relationship between raindrop diameter and rainfall intensity could be expressed as an exponential or power function relationship. Sajjadi and Mahmoodabadi [11] 1 1 carried out raindrop splashing tests under two different rainfall intensities (57 mm h− and 80 mm h− ). The results showed that the splash erosion amounts of fine particles with sizes <0.043 mm were greater than those of large particles under a higher rainfall intensity. Moreover, with increasing rainfall duration, the rate of the total splash erosion observed at the beginning of the rainfall reaches a peak value: The larger the rainfall intensity, the less time it takes to reach the peak value [12]. Ziadat and Taimeh [13] reported that soil erosion on cultivated land was primarily affected by moisture content, whereas on uncultivated land it was mostly affected by slope steepness. Lim et al. [14] suggested that a power function relationship existed between raindrop energy and rainfall intensity. Free [15] showed that the splash erosion amount was positively correlated with rainfall energy and that raindrop energy was an important index to evaluate the degree of soil erosion caused by rainfall [14]. Rainfall intensity, raindrop energy, and their variations in time and space have important effects on the prediction of rainfall erosion [16,17]. At present, most research on raindrop energy for splash erosion has used a raindrop device to simulate natural rainfall [18] and control the raindrop energy by adjusting the rainfall intensity or height [16,19–21]. However, the rainfall energy of natural rainfall simulated by a raindrop generator is instantaneous energy, which has a range of changes, and an increase or decrease in the energy has a significant impact on the amount of raindrop splash erosion. Therefore, a raindrop generator with constant raindrop energy should be used for experimental studies to ensure that the results of the study are more accurate. The splash collection device was mainly composed of an Ellison splashing pan [22], an Ellison splashing cup [23], and a Morgan splashing pan [24]. The improved device [20,25,26] could collect most soil splash erosion particles, but the horizontal spatial distribution of the splash amount and particle size have been less well studied. Furthermore, most previous studies have used dried soil [27] or disturbed soil [20,28], which destroyed the soil structure and reduced the reliability of the data. The objectives of this study were to (i) clarify the effect of raindrop energy on the splash distance and particle size distribution of aggregate splash erosion, (ii) establish a prediction equation for splash erosion in this area, and (iii) predict whether a certain raindrop energy will break up the aggregates to a great extent by comparing the characteristic parameters of the aggregate fractions. 2. Materials and Methods 2.1. Sampling Site and Soil Properties The sampling site is located in the Changwu agriculture ecological experimental station, Weibei Plateau, Xianyang, Shanxi province (107◦40059” E, 35◦14027” N), and has an annual average temperature of 9.1 ◦C and annual average precipitation of 580.0 mm. The zonal soil is dark loessial soil [29]. The soil is loose, and the permeability is good, resulting in a good “soil reservoir” effect. The international soil texture classification is loam soil according to the USDA (United States Department of Agriculture) particle size classification criteria. Twenty-one samples and 1000 g of scattered soil were collected from the top layer (0–20 cm) of cultivated land using a cutting ring (10 cm diameter 5 cm height) × and the diagonal method. Three of the samples were used to determine the soil bulk density and moisture content, and the remaining 18 samples were used for the raindrop splash experiment. After the scattered soil dried naturally, the bulk density, moisture content, soil organic matter, total nitrogen, total phosphorus, and mechanical components were determined using the cutting ring (determine the bulk density and moisture content), potassium dichromate external heating, Kjeldahl nitrogen, HClO4–H2SO4, and Malvin laser particle sizer methods. The soil characteristics of the sampling sites are reported in Table1. WaterWater 20192019,, 1111,, x 1514 FOR PEER REVIEW 3 3of of 11 11 Table 1. Physical and chemical properties of the soil. Table 1. Physical and chemical properties of the soil. Soil Particle Size Composition/% Bulk Moisture Total Total Particle Size Composition/% Soil Bulk Organic Total Total Sand Silt Soil Density ContentMoisture Soil OrganicNitrogen Phosphorus Clay (<0.002 Type Density/ Carbon Nitrogen/ Phosphorus(2–0.02/ Sand (0.02–0.002Silt Type −3 Content/% Carbon/% −1 −1 Clay /(g·cm(g cm) 3) /% /(g·kg(g) kg 1) /(g·kg(g) kg 1) (2–0.02 (0.02–0.002 mm) − /% − − mm) mm) (<0.002 mm) · · · mm) mm) 1.1 ± 0.1 18.8 ± 1.0 1.6 ± 0.1 1.0 ± 0.1 0.7 ± 0.03 52.3 ± 0.5 37.4 ± 0.03 10.3 ± 0.04 Dark 1.1 0.1 18.8 1.0 1.6 0.1Fragment 1.0 size0.1 distribution 0.7 0.03 of the undisturbed52.3 0.5 soil/%37.4 0.03 10.3 0.04 Dark ± ± ± ± ± ± ± ± Fragment size distribution of the undisturbed soil/% loessialloessial 0.5–1 >2> mm2 mm 1–2 1–2 mm mm 0.5–1 mm0.25–0.5 0.25–0.5 mm mm0.053–0.25 0.053–0.25 mm mm <0.053<0.053 mm mm soilsoil mm 6.9 1.8 12.3 1.1 21.3 4.2 21.1 2.1 14.6 4.1 23.9 2.9 6.9 ± 1.8± 12.3 ± 1.1± 21.3 ± 4.2± 21.1 ± 2.1 ± 14.6 ± 4.1± 23.9 ± ±2.9 2.2.2.2. Experimental Experimental Design Design TheThe artificial artificial rainfall rainfall device used in this studystudy consistedconsisted ofof twotwo parts:parts: AA raindropraindrop generator generator and and a asplashed splashed raindrop-collecting raindrop-collecting device device (Figure (Figure1)[ 1)30 [3].0].
Details
-
File Typepdf
-
Upload Time-
-
Content LanguagesEnglish
-
Upload UserAnonymous/Not logged-in
-
File Pages11 Page
-
File Size-