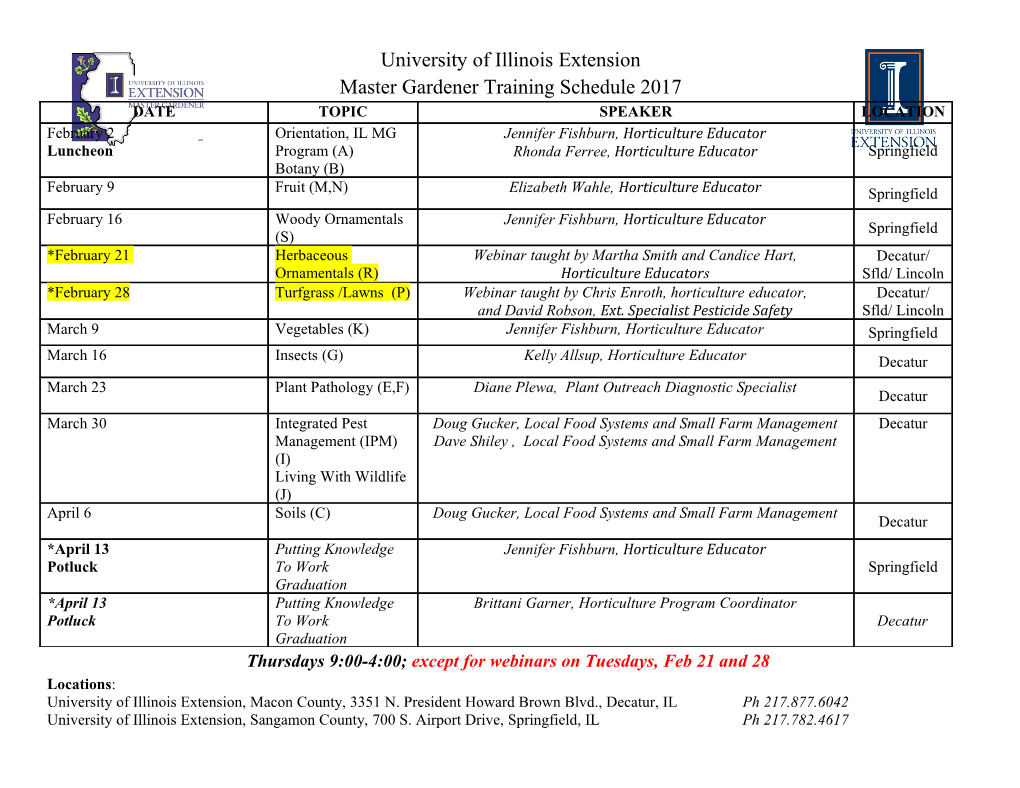
New Constraints on Precambrian Ocean Composition1 fohn P. Grotzinger and fames F. Kasting2 Department of Earth, Atmospheric and Planetary Sciences, Massachusetts Institute of Technology, Cambridge, MA 02139 ABSTRACT The Precambrian record of carbonate and evaporite sedimentation is equivocal. In contrast to most previous interpre­ tations, it is possible that Archean, Paleoproterozoic, and to a lesser extent, Meso to Neoproterozoic seawater favored surplus abiotic carbonate precipitation, as aragonite and (hi-Mg?) calcite, in comparison to younger times. Further­ more, gypsum/ anhydrite may have been only rarely precipitated prior to halite precipitation during evaporation prior to about 1.8 Ga. Two effects may have contributed to these relationships. First, sulfate concentration of seawater may have been critically low prior to about 1.9 Ga so the product mea++ · m 504 - would not have produced gypsum before halite, as in the Mesoproterozoic to modern ocean. Second, the bicarbonate to calcium ratio was sufficiently high so that during progressive evaporation of seawater, calcium would have been exhausted before the gypsum field was reached. The pH of the Archean and Paleoproterozoic ocean need not have been significantly different from the modern value of 8.1, even at C02 partial pressures of a tenth of an atmosphere. Higher C02 partial pressures require somewhat lower pH values. Introduction Precambrian Carbonates and Evaporites It is generally assumed that there have been no The Precambrian sedimentary record to about 3.5 significant changes in the composition of seawater Ga includes dolostones and limestones precipi­ during the past 3.5 Gyr (Holland 1972, 1984; tated originally as aragonite and calcite (Grotzinger Walker 1983). This is based principally on past 1989; Grotzinger and Read 1983; Hofmann and claims that the sequence of carbonate and evapo­ Jackson 1988; Knoll and Swett 1990). It is subdivis­ rite minerals has remained constant. On this basis, ible into several overlapping periods marked by sig­ limits have been placed on allowable deviations nificant variations in the quantity and mode of car­ of the concentrations of the major constituents of bonate precipitation (Grotzinger 1989). Archean seawater during the Neoproterozoic (Holland 1984) carbonate sedimentation featured prolific precipi­ and, allowing for significantly higher C02 pres­ tation of aragonite as giant botryoids up to 1 m in sures, the Archean (Walker 1983). However, the radius and magnesian calcite as stratigraphic model for constant seawater composition recently sheets up to several meters thick and extending has been challenged on an observational (Grot­ along strike for over 100 km, in addition to a vari­ zinger 1989) as well as theoretical basis (Kempe ety of stromatolite types (figure 1 ). By comparison, and Degens 1985). Here, we discuss the signifi­ Paleoproterozoic carbonate sedimentation was cance of non-uniformitarian carbonate and evapo­ marked by less spectacular occurrences of mas­ rite mineral precipitation. Previous constraints on sively precipitated aragonite and calcite, although the composition of seawater are revised in light of sedimentation by precipitation of cement crusts, this new evidence that help define likely unidirec­ particularly in tidal flat settings as microdigitate tional changes in the C02 pressure of the atmo­ stromatolites, remained a characteristic mode of s·phere and tectonic regime of the early earth. platform aggradation (figure 2). In contrast, Meso­ through Neoproterozoic carbonate sedimentation saw the progressive decline in precipitation of mas­ sive carbonate cements, although precipitation as 1 Manuscript received June 15, 1992; accepted December 1, 1992. cryptocrystalline micritic whitings was typical, 2 Department of Geosciences, Pennsylvania State Univer­ particularly in the Neoproterozoic (Knoll and sity, University Park, PA 16802. [The Journal of Geology, 1993, volume 1_01, p. 235-243] © 1993 by The University of Chicago. All rights reserved. 0022-1376/93/10102-010$1.00 235 236 JOHN P. GROTZINGER AND JAMES F. KASTING AGE (Ga) AGE (Ga) 0.6 0.6 0.8 I 0.8 I Neoproterozoic Neoproterozoic Mesoproterozoic Meso proterozoic 1.0 1.0 I M 1.2 1.2 I 1.4 1.4 L 1.6 1.6 Meso proterozoic Meso proterozoic 1.8 Paleoproterozoic 1.8 Paleoproterozoic I I K I J •G • I 2.0 H 2.0 I H I G I F E 2.2 2.2 I D 2.4 2.4 Paleoproterozoic Paleoproterozoic I Archean Archean 2.6 E I 2.6 1 I I I I F A 8 C c D 2.8 Figure 2. Temporal distribution of microdigitate stro­ matolites (tidal-flat marine cement crusts). The bar 3.0 I • A B length is the error in age of the stratigraphic unit. Note Figure 1. Temporal distribution of massive, thick beds the temporal decrease in the importance of this facies. of marine cements. This includes botryoidal calcite (for­ Each bar represents a different basin; only one bar is merly aragonite) as well as herringbone calcite (formerly tabulated no matter how many beds occur within the Mg-calcite) that form discrete beds greater than 1 m basin. If individual beds were tabulated then the high thick. The bar length is the error in age of the strati­ frequency in the Paleoproterozoic would appear even graphic unit. Note the temporal decrease in the impor­ greater. Sources are provided in figure 15 of Grotzinger tance of this facies. Each bar represents a different basin; (1989). only one bar is tabulated no matter how many beds occur within the basin. If individual beds were tabulated, the high frequency in the Archean would appear even itation of calcite and aragonite cements directly on greater. Sources: A (Hofmann et al. 1985); B (Hofmann the seafloor is only known from rare intervals in 1971 ); C (J. Grotzinger and N. Beukes, unpub. data); D the Phanerozoic, such as the Permian (Yurewicz (Martinet al. 1980); E (Sumner et al. 1991 ); F (Simonson 1977). It seems probable therefore that Precam­ et al. 1993); G (Hofmann and Grotzinger 1985); H (Grot­ brian seawater was greatly oversaturated with re­ zinger and Friedman 1989); I (Peryt et al. 1990). spect to calcium carbonate and that saturation de­ creased to Phanerozoic levels throughout the Swett 1990). The Neoproterozoic was a transi­ Proterozoic (Grotzinger 1989). This long-term de­ tional interval; certain facies were similar to ear­ crease in saturation likely involved numerous lier Proterozoic sequences, but others were akin to shorter-term oscillations when the trend may have Phanerozoic equivalents. been locally reversed. However, data are so sparse Today, it is significant that despite the 3- to 7- that it is not possible to delineate anything other fold oversaturation of modern surface seawater than the billion-year-scale trend. with respect to calcium carbonate (Li et al. 1969), There is considerable evidence for Precambrian precipitation of massive seafloor cements is un­ evaporite sedimentation. Evidence for halite is known; all known modern marine cements are re­ present throughout the record and for the earlier stricted to filling pores in reefal buildups or uncon­ Precambrian, is developed where gypsum is absent. solidated debris. Biological secretion accounts for In the late Archean (ca. 2. 7 Ga) Fortescue basin, almost all carbonate precipitation in the modern Western Australia, Buick (1992, p. 76) has de­ ocean, despite the fact that the ocean is still sig­ scribed "curtailed evaporite paragenesis, from car­ nificantly oversaturated. Similarly, massive precip- bonate straight to halite without an intervening Journal of Geology CENTENNIAL SPECIAL ISSUE 237 stage of sulfate precipitation." Because the precipi­ tation sequence differs from Phanerozoic marine evaporites, Buick (1992) interprets it as evidence for lacustrine sedimentation. However, there are no independent "diagnostic" criteria supporting this inference, and a marine origin cannot be dis­ counted. If it is marine, this sequence suggests that BLANCHET the composition of seawater was substantially dif­ ferent at that time. A second example of carbonate­ to-halite precipitation is found in the 1.90 Ga BACK·REEF FACIES Rocknest Formation, Northwest Canada. The ~0010 RAMP/FORESLOPE FACIES ~ GRAINSTONE Rocknest Formation is dominated by restricted DOUGLAS ~COLUMNAR ~LIMESTONE ~MICROBIAL tidal-flat facies containing halite casts with no ~LAMINITE BASINAL FACIES EJ~~:~E evidence of former gypsum or anhydrite despite t==::=l LIMESTONE ~IJOMAL E;:3 RHYTHMrrE ~STROMATOLITE sedimentological (Grotzinger 1986) and isotopic REEF FACIES ~COLUMNAR ~ STROMATOLrrE MOUNDS/ lilir::J:I COLUMNAR (Burdett et al. 1990) evidence for widespread evapo­ ~ MARLSTONE ~ GRAINSTONE CHANNELS ~ STROMATOlrrE rative conditions. A third example of an evaporite EVAPORITE COLLAPSE BRECCIA sequence that proceeded directly from carbonate to halite occurs in the ca. 1.88 Ga Pethei Group (East Figure 3. Stratigraphic cross-section of Pethei Group Great Slave Lake, Canada). The Pethei Group rep­ and overlying Stark Formation (megabreccia). Note well­ resents a major, shallow-water carbonate platform developed paleogeographic zonation of carbonate facies, and adjacent basin (Hoffman 1974). The uppermost strong platform-to-basin differentiation, and formation formations, Hearne and Pekanatui, represent the of evaporite-collapse breccia over basinal as well as shal­ final stages of carbonate precipitation in respec­ low-water carbonate facies. After Hoffman (1974) and tively shallow- and deep-water environments (fig­ Hoffman et al. (1977). The Pethei platform is developed ure 3). These formations are abruptly overlain by on a spatial scale comparable to the largest Phanerozoic platforms and likely faced a major ocean. It is very diffi­ over 100m of megabreccia (Stark Formation) inter­ cult to reconcile the passage of carbonate precipitation preted to have formed by evaporite dissolution and directly into halite precipitation unless the composition collapse (Hoffman et al. 1977). The breccia consists of that ocean was changed relative to younger times. For of chaotically dispersed blocks of stromatolitic car­ further elaboration, see text and figure 25.5 in Hoffman bonate up to SO by 1000 m, dispersed in a brecci­ et al. (1977). ated matrix of red mudstone. Hoffman et al. (1977, p.
Details
-
File Typepdf
-
Upload Time-
-
Content LanguagesEnglish
-
Upload UserAnonymous/Not logged-in
-
File Pages9 Page
-
File Size-