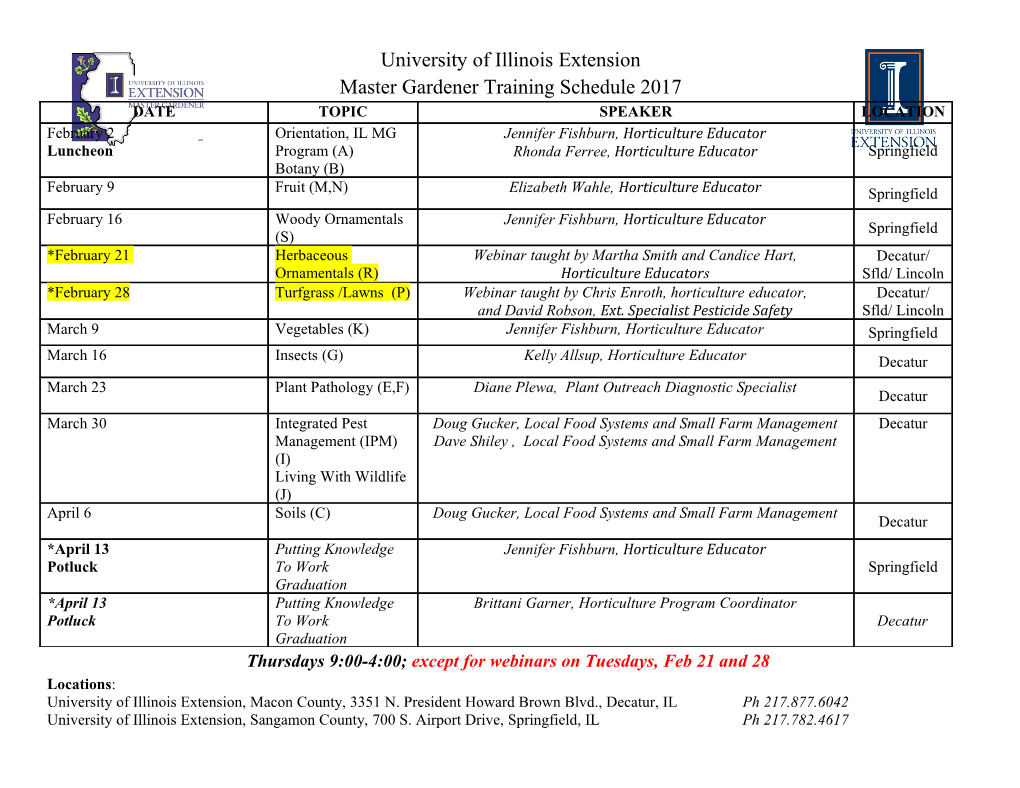
The Pennsylvania State University The Graduate School Department of Chemistry THE SYNTHESIS AND CHARACTERIZATION OF FUNCTIONALIZED NANOTHREADS A Thesis in Chemistry by Daniel Koeplinger © 2019 Daniel Koeplinger Submitted in Partial Fulfillment of the Requirements for the Degree of Master of Science May 2019 ii The thesis of Daniel Koeplinger was reviewed and approved* by the following: John V. Badding Professor of Chemistry, Physics, and Materials Science and Engineering Thesis Advisor John B. Asbury Associate Professor of Chemistry Ben J. Lear Associate Professor of Chemistry Philip C. Bevilacqua Distinguished Professor of Chemistry, Biochemistry and Molecular Biology Head of the Department of Chemistry *Signatures are on file in the Graduate School. iii ABSTRACT Carbon nanothreads are a relatively new type of carbon material which has an sp3 structure similar to diamond, but extends in only one dimension. The predicted properties of nanothreads are extraordinary, rivaling other recent carbon materials such as carbon nanotubes and graphene in both strength and stiffness. Nanothreads are synthesized experimentally by slow compression of benzene to about 20 GPa, followed by slow decompression, resulting in a crystalline product in which the polymer is arranged into a hexagonally close-packed structure. The material can be identified via its characteristic six-fold diffraction pattern, as well as its Raman and IR spectra. In addition to nanothreads produced from benzene, several new types of nanothreads have also been synthesized from other precursors, such as pyridine and aniline. In Chapter 2.1 of this work, experiments involving the compression-induced polymerization of three additional aromatic molecules are described. Toluene produced the most promising results, yielding a transparent amorphous solid which has similar IR and Raman spectra to those of previous nanothreads. Nanothread syntheses were also attempted using benzoic acid and hexabromobenzene as precursors, but no significant reaction was seen for either material. Nanothread structure is complex, and there are many structures of similar energy which are thermodynamically possible. In addition to the fully-saturated sp3 products from a Degree-6 polymerization, products which result from incomplete polymerization, including unsaturated Degree-4 and Degree-2 segments, are observed. Each of these structures has varying amounts of sp2 character. A series of experiments are described in Chapter 2.2 in which the ratio of sp2 to sp3 bonds in the nanothread product was measured via advanced solid-state NMR. These ratios were used to approximate the relative quantities of Degree-2, -4, and -6 structures for both benzene iv and pyridine nanothreads. NMR data was also used to constrain which isomers of each Degree might be found in experimentally-synthesized nanothreads. When nanothreads are formed under pressure, they produce crystals of close-packed fibers. These fibers are bound together primarily by Van-der-Waals forces, and application of mechanical force could theoretically exfoliate individual threads from the bulk. In Chapter 3, exfoliation experiments are described in which three separate methods attempted to separate individual threads from the bulk: applying shearing forces by twisting samples between two planes, peeling fibers away from the bulk using tape adhesive, and crushing the nanothread crystals with the tip of a diamond. Samples from each of these methods were examined by atomic force microscopy. Identification of individual fibers separated from the bulk nanothread crystal proved problematic because of the small radii of nanothreads, particularly when compared to the resolution of techniques used to characterize nanothreads in the bulk. As a result of these limitations, the only way to prove that a fiber was a nanothread would be to find a thread still partially attached to the bulk. No partially-attached fibers were observed by AFM using the three preparation methods listed above, but the possibility of identifying such a feature in the future was not excluded. v TABLE OF CONTENTS List of Figures ………………………………………………………………....………………... vi List of Tables …………………………………………………………………....…....……..….. ix List of Abbreviations ……………………………………………………………………...…...... x Acknowledgments …………………………………………………………......……….…….... xii CHAPTER 1 Introduction ……………………………………………………………………...... 1 1.1 Computational Predictions of Structure and Properties 1.2 Synthesis of Benzene Nanothreads 1.3 Synthesis of Other Nanothreads 1.4 Potential Applications for Nanothreads CHAPTER 2 Use of Benzene Derivatives and Isotopes in Synthesis ……..………………...… 17 2.1 Synthesis of Nanothreads from Benzene Derivatives 2.2 Solid-State NMR Studies on 13C Benzene and 15N Pyridine Nanothreads CHAPTER 3 Mechanical Exfoliation of Benzene Nanothreads ………………………............. 41 CHAPTER 4 Conclusions and Future Outlook ………………………………………………... 49 References ………………………………………………………………………...….………… 50 Appendix: Additional Copyright Information …………………………………………………. 54 vi LIST OF FIGURES Figure 1: (a) Computationally predicted stress-strain curves for three nanothread isomers, which predict the relative strength and stiffness of each. (b-d) Diagrams of each of isomer with each bond colored according to its stress near the ultimate tensile strength of its structure. Adapted with permission from [5] Zhan, et al. 2016…………………………………………….…….….. 5 Figure 2: Example structures of each possible nanothread Degree, including Degree-0, which is simply an organized stack of benzene rings which are not bonded to one another. Adapted with permission from [8] Chen, et al. 2017……………………………………………...………....…. 7 Figure 3: A TEM micrograph of one of the original nanothread products showing ordered striations about 6.4 Å apart. Reprinted with permission from [9] Fitzgibbons, et al. 2014.…..… 9 Figure 4: (a) Models of polytwistane arranged into hexagonal packing structures, demonstrating the 6.5 Å distance between threads and the 5.6 Å interplanar spacing. (b) A six-fold x-ray diffraction pattern resulting from diffraction in the c-axis of the crystal. (c) A two fold x-ray diffraction pattern resulting from diffraction in the b-axis. Reprinted with permission from [13] Li, et al. 2017………………………………………………………………………………….... 10 Figure 5: Sketch of a diamond anvil cell, as used in a typical nanothread synthesis. A sample is enclosed inside of a gasket between two diamonds. These diamonds are then pressed together, concentrating force into the tips (culets) of the diamonds. This generates extreme pressures within the sample, which is used as the driving force behind nanothread polymerization…….. 11 Figure 6: Raman spectra for a sample of toluene excited at a wavelength of 532 nm under increasing amounts of pressure within a DAC. The starting pressure was 4.56 GPa, moving upward to 22.97 GPa, where the photoluminescent background overtook the samples. All subsequent measurements during the remainder of the compression and decompression showed only an elevated background with no significant peaks…………………………………...…… 19 Figure 7: The mid-IR absorption spectrum of (Blue) the toluene compression product and of (Red) a sample of benzene nanothreads………………………………………………………… 21 Figure 8: Image of the toluene compression product (darker center circle) in its stainless steel DAC gasket (bright outer ring). Image captured on an Olympus BX62 Microscope in reflection mode…………………………………………………………………………………………….. 23 Figure 9: Raman spectra for a sample of HBB excited at a wavelength of 532 nm under increasing amounts of pressure within a DAC. The starting pressure was 3.73 GPa, moving upward to 25.11 GPa. The sample was then decompressed and the recovered sample was measured at ambient pressure…………………………………………………………………... 29 Figure 10: The Raman spectra from 0-1800 cm-1 of (Blue) the HBB Compression Sample and (Red) a sample of unmodified HBB, each excited at a wavelength of 514 nm. The wavelengths of peaks common to both of the samples are labeled. (Green) The Raman spectrum of a sample of benzene nanothreads excited at 633 nm is included for comparison………………………... 30 vii Figure 11: Reaction scheme for the proposed monomer chain transfer reaction in HBB para polymer, a Degree-2 nanothread. In Step (a), a bromine radical is eliminated in the position para one of the end radicals, leaving a fully aromatic chain-end residue with five remaining bromides which will no longer grow unless it comes in contact with the growing end of another chain. In Step (b) the bromine radical reacts with an HBB monomer to produce either (1) a Br2 molecule plus a new growing chain with an identical chain-end residue from the one produced in Step (a), or (2) a new growing chain with a chain end residue containing seven bromides. A similar monomer chain transfer reaction would be expected for a diradical-mediated 4+2 reaction…... 35 Figure 12: The 13C NMR spectra of 13C-enriched benzene nanothreads. (a) The proton-decoupled multiCP spectrum of all carbons in the sample, which allows calculation of the relative prevalence of sp2- and sp3-hybridized carbon in the sample. (b) The dipolar DEPT spectrum, giving signal only from CH features. This is compared to the dipolar DEPT spectrum of amorphous polystyrene (PS). (c) The three-spin coherence spectrum, giving signal only from CH2 features. (d) The dipolar dephased multiCP spectrum, giving signal only from non- protonated or mobile carbon. Reprinted with permission from [42] Duan, et al. 2018.………... 37 Figure 13: The percentage of carbon atoms
Details
-
File Typepdf
-
Upload Time-
-
Content LanguagesEnglish
-
Upload UserAnonymous/Not logged-in
-
File Pages67 Page
-
File Size-