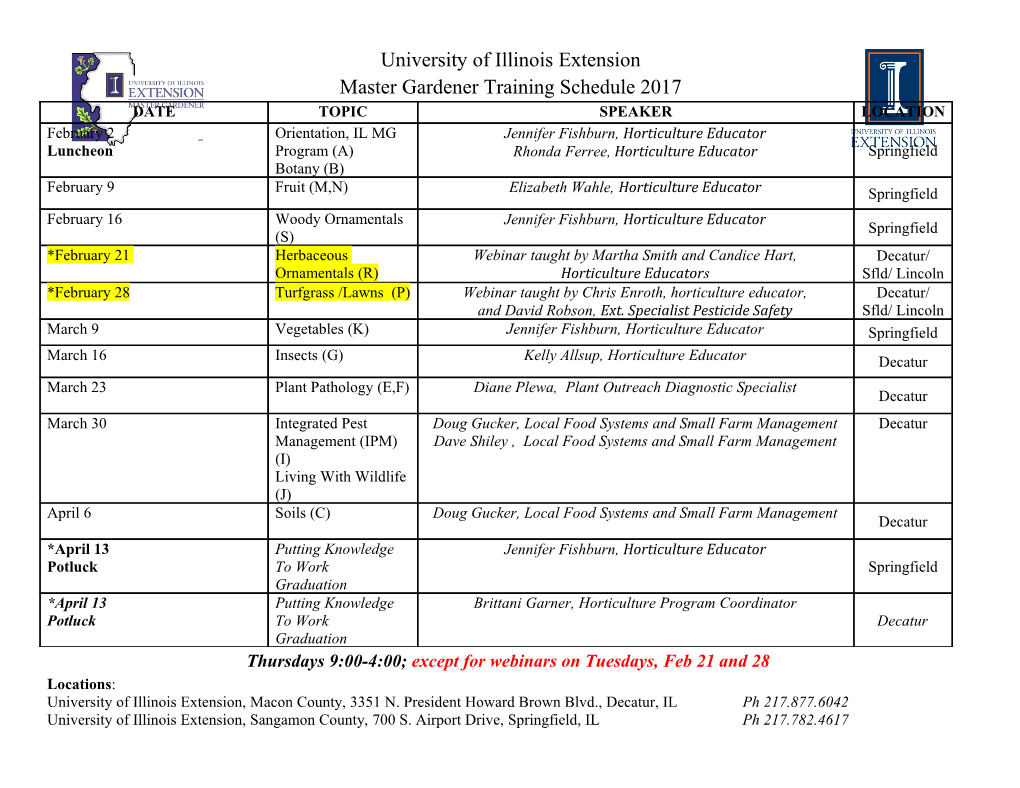
Computational Chemistry in Organic Chemistry Lecture using WebMO Brian J. Esselman,* Aubrey J. Ellison,* and Jia Zhou Department of Chemistry, 1101 University Avenue, Madison, WI 53706 5 ABSTRACT Advances in computational software and hardware have allowed computational chemistry to become a more integrated component of undergraduate organic chemistry curriculum. With a few exceptions, most of this attention has been given to small lecture activities or individual laboratory exercises. To allow students to fully utilize the insights of computational chemistry, it must become 10 fully integrated into all aspects of instruction. Here we present out on-going efforts in integrating computational chemistry into the entire lecture curriculum via carefully chosen examples on lecture slides, problem sets, and assessments. Rather than having students perform these calculations themselves, as they do in our laboratory course, we have taken advantage of the HTML-export feature of WebMO to provide students with direct links to computational data. As students work through our 15 curriculum, their learning is supported by the ability to view relevant molecular geometries, charge distributions, orbitals, vibrational modes, etc. We are confident that use of these tools leads to better learning outcomes. INTRODUCTION Coinciding with the availability of student-friendly software, computational chemistry has been 20 introduced in many graduate and undergraduate courses over the past few decades.1-7 Many excellent examples of lecture and laboratory exercises have been presented in the literature across college-level instruction. Given the obvious ability of computational chemistry to help students understand and visualize the three-dimensional nature of molecules and highlight the connection between structure and reactivity, it is unsurprising that instructors of general, organic, and inorganic chemistry have 25 seized upon this capability. Importantly, computational results can provide chemical insights to students without the need to understand the details of how the calculations were performed. Hence, many published exercises designed for early undergraduate chemistry students are able to avoid 1 delving deeply into the details of quantum mechanics and computational chemistry. This demonstrates the ability of computational results to provide chemical insights to students without the 30 need to understand the details of how the results were obtained. While there is definitely substantial value in a proper understanding of quantum mechanics, levels of theory, basis sets, optimization routines, etc., these topics can be reserved for upper-level physical chemistry and computational chemistry courses without sacrificing substantial student understanding of structure and bonding in the introductory courses. 35 Stand-alone computational chemistry exercises have the inherent drawback of time, cognitive load, and effort required to learn how to perform the calculations. This burden, in cases of infrequent implementation, can easily overshadow the insights derived from the exercise that would be useful to the early college student. A few university courses8-10 and department-wide chemistry programs11-13 have embedded computational chemistry throughout the curriculum. These implementations reduce 40 the cognitive load on students in any particular computational exercise as students become increasingly familiar with the software due to repeated exposure. Additionally, students and instructors inherently see the benefit of mastering the new software necessary to complete their calculations, knowing that this skill will serve them well in their future coursework. Undoubtedly, such repeated utilizations can provide a more meaningful and longer-lasting impact on students’ 45 understanding of three-dimensional structures and chemical bonding. In organic chemistry, there are two very natural avenues for computational chemistry to support the curriculum: supporting analysis of experimental data and elucidating how the electronic or molecular structure drives the chemical outcome. In the organic laboratory, many authors have demonstrated that computational chemistry can help students assign IR or NMR spectra.4, 14-22 This 50 support can allow students to make assignments and perform analyses that may be beyond their abilities without computational predictions. Further, a number of authors have demonstrated the utility of calculations to help students predict or rationalize the outcome of an organic reaction in lecture and laboratory settings,23-26 helping students obtain a deeper understanding of many important concepts. Student exercises have been used to investigate conformational isomerism,9-10, 27- 55 31 structure and relative energy of reactive intermediates,19, 21, 25-26, 32-37 the selectivity of an organic 2 reaction,19, 25-26, 38-42 assessing relative acidity,43-44 evaluation of a reaction mechanism,45 etc. All of these examples provide other educators a template for how they can enhance their curricula and lead to better student learning. We wish to take this process one step further and integrate computational outputs throughout the 60 entire organic lecture curriculum. Focusing on interpreting computational results will provide the benefits of a deeper conversation about structure, bonding, and reactivity, without substantially adding to the cognitive load and time necessary for students to perform the calculations. Based upon previous work,30, 46-47 we have embedded WebMO7-HTML exports into our course materials: student handouts, problem sets, lecture slides, discussion activities, and exams. In a manner similar to 65 Springer,46 we present computationally generated images of molecules alongside more common two- dimensional images in lecture. We have extended this use of three-dimensional images from WebMO to include all course content, allowing students to view structures and orbitals in a virtual model kit. Alongside these computer-generated structures, we encourage students to use wedge-dash notation for molecules drawn on paper and traditional model kits to help build their representational 70 translation. While we have just begun this modernization of our curricular materials, we intend to make virtually all images of molecules presented in course content accessible via student engagement with HTML links. Even during this early adaptation and implementation, we have observed several important anecdotal outcomes. Our students seem to have a deeper grasp of three-dimensional structures, an improved understanding of how atomic and molecular orbitals influence chemical 75 reactivity, and a greater mechanistic focus on why and how reactions occur. As an intended consequence, students are able to move away from pattern recognition and memorization and toward utilizing key concepts to rationalize reactivity. We have changed our own teaching frame of mind to be more focused on how the molecular and electronic structure leads to the function of the molecule. This article presents some of our initial work and reflections. 80 CURRICULAR IMPLEMENTATION The representative examples shown here are from 1st and 2nd semester organic chemistry lectures (CHEM 343 and CHEM 345) at the University of Wisconsin–Madison. This is the main sequence of 3 organic chemistry and has between 200 and 300 students per lecture in a wide range of majors. Together, these courses cover the topics presented in the 6th edition of Organic Chemistry by Loudon 85 and Parise.48 The course does not have a laboratory component, and students may take the laboratory course (CHEM 344) concurrently with or subsequent to CHEM 345. Students may have some exposure to computational chemistry through use of computational outputs in general chemistry or later in CHEM 344 where they run calculations to support their understanding of molecular structure and properties.9-10 Regardless of their level of exposure to output files prior to CHEM 343, 90 virtually none of these students have used any computational chemistry software to perform calculations themselves. These representative examples demonstrate the usage of WebMO-HTML exports in lecture notes, problem sets, and on course assessments. Each figure, in the following section, is an authentic image of computational results used in our courses and instruction to support student learning. These figures include examples of geometry optimizations, vibrational frequencies, 95 NMR chemical shift predictions, and Natural Bond Orbital (NBO) calculations. The NBO calculation generates and visualizes the molecular orbitals and natural bond orbitals; we use whichever is most impactful for illustrating a concept to students. In general, MOs are useful for depicting conjugated systems as MOs inherently show the delocalization of electrons throughout a molecule. NBOs are useful for depicting lone pairs and bonds using the hybridizations and localized notions of bonding 100 common in organic and general chemistry. 1st semester examples Starting with the very first lecture, students are introduced to computational depictions of chemical structures. The early introduction is done in such a way that computational models are intentionally presented in parallel with Lewis structure drawings. This pairing serves to build student 105 confidence and understanding in translating two-dimensional representations into accurate chemical geometries, as well as to demonstrate the utility of computational models. Figure 1 shows the WebMO output of methane embedded directly
Details
-
File Typepdf
-
Upload Time-
-
Content LanguagesEnglish
-
Upload UserAnonymous/Not logged-in
-
File Pages21 Page
-
File Size-