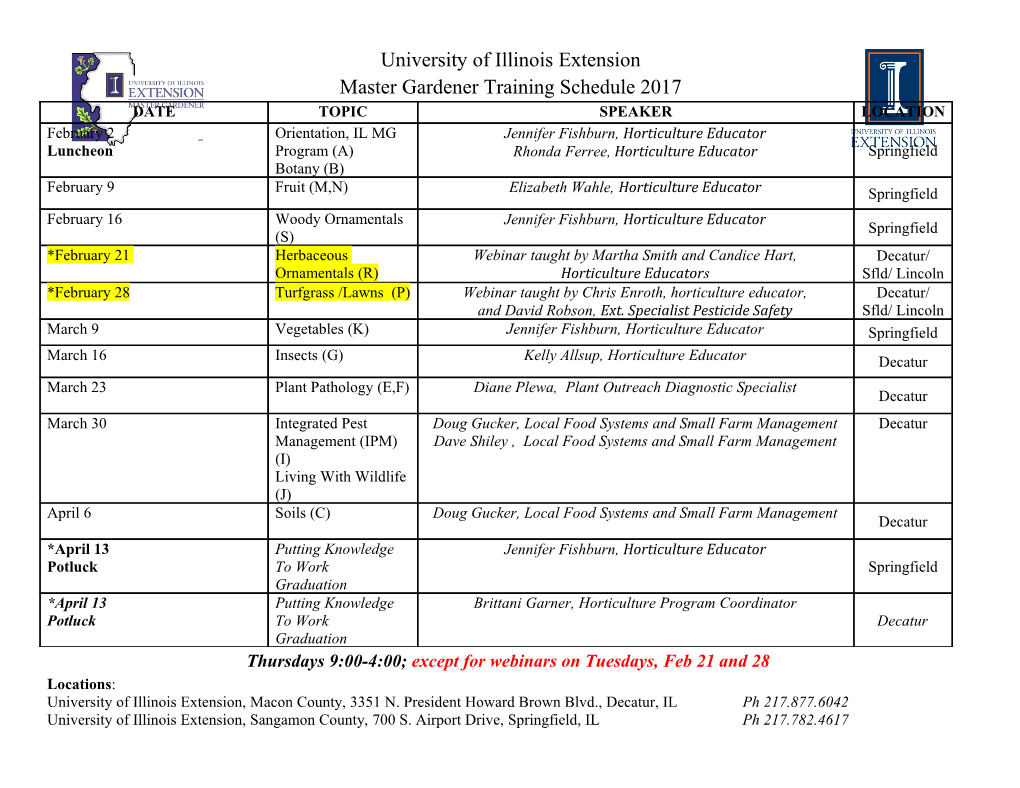
Lithium Amide Halides for Hydrogen Storage by Rosalind Davies Supervisor: Dr. Paul Anderson A thesis submitted to The University of Birmingham for the degree of Doctor of Philosophy Centre for Hydrogen and Fuel Cell Research School of Chemical Engineering University of Birmingham B15 2TT University of Birmingham Research Archive e-theses repository This unpublished thesis/dissertation is copyright of the author and/or third parties. The intellectual property rights of the author or third parties in respect of this work are as defined by The Copyright Designs and Patents Act 1988 or as modified by any successor legislation. Any use made of information contained in this thesis/dissertation must be in accordance with that legislation and must be properly acknowledged. Further distribution or reproduction in any format is prohibited without the permission of the copyright holder. Abstract The lithium amide halides are a promising series of materials for hydrogen storage as they release hydrogen at a lower temperature than lithium amide on reaction with lithium hydride. The amide chloride system has been studied in detail, and a phases with reduced chloride content, of stoichiometry Li7(NH2)6Cl, was identified by powder X-ray diffraction and Ra- man spectroscopy. This phases was seen to release hydrogen on reaction with LiH at a lower temperature than observed for lithium amide, and ammonia release was suppressed. The material was also able to be cycled, with the chloride ions maintained within the structure during desorption and rehydrogenation. The rehydrogenation of the imide dehydrogenation products of the new phase occurred more readily under the conditions used than for the known phase Li4(NH2)3Cl. The hydrogen cycling properties of Li7(NH2)6Cl were investigated alongside the amide bromide Li7(NH2)6Br and the amide iodide Li3(NH2)2I. The systems successfully cycled hydrogen, and the reversible structural changes that happened during cycling were studied. All three materials, however, showed a capacity loss on cycling under dynamic vacuum. Bulk cation transport has been identified as a key factor in the release and uptake of hydrogen in the Li-N-H system. The conductivity of the amide and imide halides was studied using A.C. impedance and found to be higher than for LiNH2 and Li2NH, respectively. This supports kinetic analyses that indicate ion diffusion is not rate-limiting for the hydrogen cycling of these systems. 1 Contents 1 Introduction 8 1.1 Hydrogen Production . 9 1.1.1 Hydrocarbon Fuel Processing . 9 1.1.2 Electrolysis of Water . 10 1.1.3 Other methods . 11 1.2 Hydrogen as a Fuel . 11 1.2.1 Internal Combustion Engine . 12 1.2.2 Fuel Cells . 12 1.3 Hydrogen Storage . 14 1.3.1 Molecular Hydrogen . 15 1.3.1.1 Compressed Gas . 15 1.3.1.2 Liquid . 15 1.3.1.3 Cryo-compressed Hydrogen . 16 1.3.1.4 Physisorption . 16 1.3.2 Metal Hydrides . 17 1.3.2.1 Magnesium hydride . 18 1.3.2.2 Aluminium hydrides . 19 1.3.2.3 Borohydrides . 20 2 1.3.2.4 Amides . 21 1.3.2.5 Mixed Lithium Magnesium Amide Systems . 23 1.3.2.6 Other Mixed Complex Hydride Systems . 25 1.3.2.7 Halide Doping in Hydrogen Storage . 25 1.3.2.8 Challenges . 27 1.4 Project Objectives . 28 2 Experimental 30 2.1 Solid state synthesis under inert gas . 30 2.2 Crystallography and diffraction . 31 2.2.1 Crystallography . 31 2.2.1.1 Crystal structures . 31 2.2.1.2 Lattice planes . 32 2.2.2 X-ray diffraction . 33 2.2.2.1 X-ray generation . 34 2.2.2.2 Monochromation . 34 2.2.2.3 Detection . 35 2.2.2.4 Laboratory X-ray diffraction . 35 2.2.2.5 Synchrotron X-ray diffraction . 36 2.2.3 Neutron diffraction . 36 2.2.4 Powder diffraction . 38 2.3 Rietveld analysis . 39 2.3.1 Quantitative phase analysis . 41 2.4 Mass spectrometry . 43 2.4.1 Ionisation . 43 2.4.2 Separation . 43 3 2.4.3 Detection . 44 2.5 Temperature programmed desorption . 44 2.5.1 Calibration . 45 2.6 Hydrogenation . 47 2.7 Raman spectroscopy . 48 2.8 A.C. impedance spectroscopy . 51 2.9 Volumetric analysis . 53 2.10 Hydrogen cycling at the Diamond Light Source . 54 2.11 Deuterium cycling on the POLARIS beamline at the ISIS neutron source . 55 2.12 Gravimetric measurements during deuterium cycling on the POLARIS beam- line at the ISIS neutron source . 56 3 Structural investigation of Li4(NH2)4 – xClx 59 3.1 Introduction . 59 3.2 Experimental . 61 3.3 The effect of composition on the kinetic product . 62 3.4 Time dependence of the formation of Li4(NH2)3Cl . 67 3.4.1 In situ monitoring of the effect of reaction time . 68 3.5 Effect of changing the Cl – composition: 12 hour reaction time . 71 3.5.1 Unknown phase . 74 3.5.2 Structural refinement of the new phase with lower chloride content . 75 3.5.3 Diamond and ISIS structural refinement . 76 3.5.4 Raman spectroscopy . 79 3.6 Time dependence of the formation of Li7(NH2)6Cl . 80 3.7 Effect of magnesium on the structure . 81 3.7.1 The effect of reaction time . 82 4 3.7.2 Raman spectroscopy . 84 3.8 Conclusions and further work . 85 4 Hydrogen storage properties of Li7(NH2)6Cl and Li6Mg1/2(NH2)6Cl 86 4.1 Introduction . 86 4.2 Experimental . 87 4.3 Dehydrogenation of Li7(NH2)6Cl and Li7Mg1/2(NH2)6Cl on reaction with LiH 88 4.3.1 Characterisation of the dehydrogenated products . 89 4.4 TPD-MS of a lithium amide/ lithium hydride/ lithium chloride mixture . 92 4.5 Rehydrogenation of the imide chlorides . 94 4.5.1 Rehydrogenation under different pressures . 97 4.6 Dehydrogenation of Li7(NH2)6Cl with a mixture of LiH and MgH2. 101 4.6.1 Hydrogenation after reaction with a LiH/MgH2 mixture . 104 4.7 Cycling properties . 105 4.7.1 TPD of rehydrogenated products . 105 4.7.2 HTP of Li7(NH2)6Cl . 107 4.7.3 Hydrogen cycling of Li7(NH2)6Cl studied using synchrotron powder X-ray diffraction on the I11 beamline . 109 4.7.4 Deuterium cycling of Li13(ND)6Cl measured by powder neutron dif- fraction . 115 4.7.5 Ex-situ characterisation of the sample after cycling . 123 4.7.6 Gravimetric cycling of Li7(ND2)6Cl studied using powder neutron diffraction . 125 4.8 A.C. Impedance Spectroscopy . 131 4.8.1 Li7(NH2)6Cl . 131 4.8.2 Li13(NH)6Cl . 134 5 4.9 Conclusions and further work . 136 5 Hydrogen storage properties of other amide halides 140 5.1 Introduction . 140 5.2 Experimental . 141 5.3 The lithium amide bromide, Li7(NH2)6Br . 142 5.3.1 Structure . 142 5.3.2 A.C. Impedance Spectroscopy . 144 5.4 TPD-MS of a lithium amide/ lithium bromide mixture . 148 5.4.1 Deuterium cycling of the bromide system measured by powder neut- ron diffraction . 151 5.4.1.1 New Br phase . 154 5.4.2 Ex-situ characterisation of the sample after cycling . 155 5.4.3 Hydrogen cycling studied by synchrotron radiation . 156 5.4.4 Deuterium cycling and gravimetric measurements . 161 5.4.5 New phase in the lithium amide bromide system . 165 5.5 The lithium amide iodide, Li3(NH2)2I . 167 5.5.1 TPD-MS of a lithium amide/ lithium iodide mixture . 167 5.5.2 Hydrogen cycling of Li3(NH2)2I studied by synchrotron radiation . 169 5.5.3 Deuterium cycling and gravimetric measurements . 173 5.5.4 Further deuterium cycling and gravimetric measurements . 178 5.6 Incorporation of fluoride . 182 5.6.1 LiNH2 + LiF . 182 5.6.2 LiNH2 + KF . 182 – 5.6.3 BF4 ................................. 182 5.7 LiHxF1 – x solid solution . 184 6 5.7.1 Hydrogen desorption . 184 5.8 Conclusions and further work . 186 6 Conclusions 188 7 Chapter 1 Introduction Currently, the majority of electrical and thermal energy is generated by the combustion of fossil fuels: coal, oil and natural gas.1 Fossil fuels are highly calorific and convenient because they have been easy to mine, transport, store and burn. However, increasing concerns about the security and longevity of their supply and the pollution caused by their combustion are leading to the need for development of alternative technologies. Air quality is another mo- tivator for alternative forms of energy production as the UK has breached the EU Air Quality Directive and, as a consequence, the Government is now subject to infraction proceedings.2 Hydrogen has the potential to form part of a renewable and sustainable energy system as an energy carrier. This is because it can be produced from renewable sources, stored and then used, with only water being emitted at the point of use. Electricity is the main alternative to hydrogen for this purpose, with batteries being used for storage. The dominance of one of these technologies over the other will depend on a number of factors. The method of hydrogen production will determine the overall.
Details
-
File Typepdf
-
Upload Time-
-
Content LanguagesEnglish
-
Upload UserAnonymous/Not logged-in
-
File Pages219 Page
-
File Size-