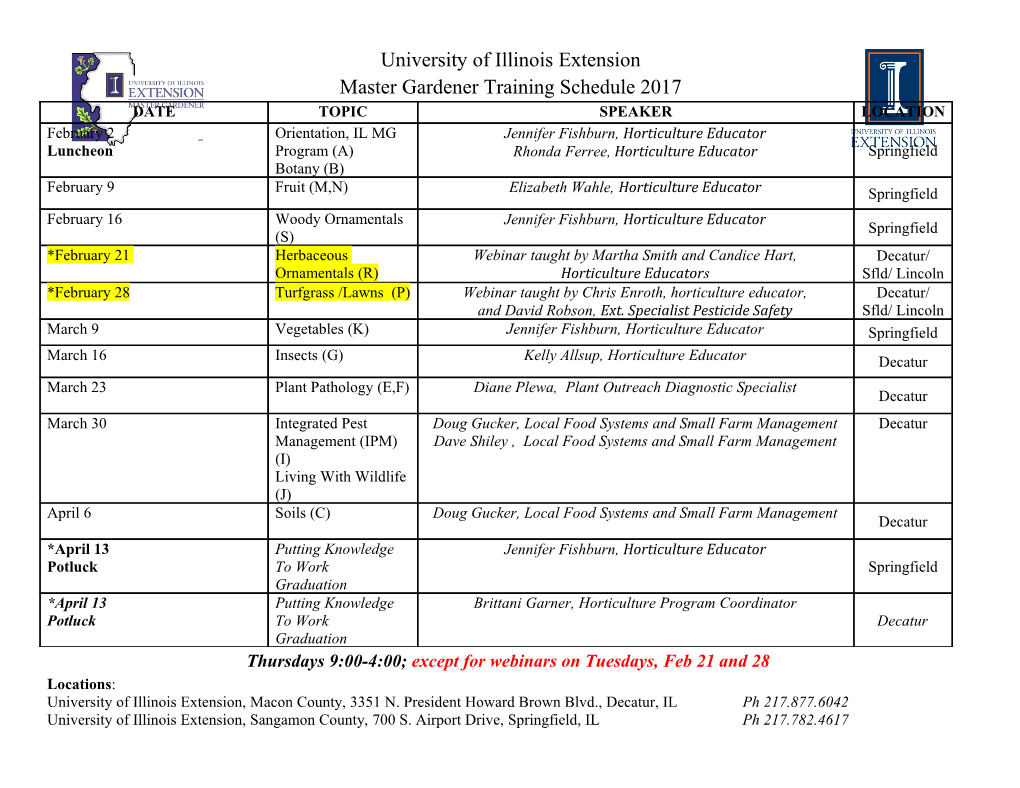
2 The Origin and Evolution of Saturn, with Exoplanet Perspective Sushil K. Atreya, Aurélien Crida, Tristan Guillot, Jonathan I. Lunine, Nikku Madhusudhan, and Olivier Mousis faceted approach that combines diverse sets of Copyright Notice observations on volatile composition and abundances, relevant properties of the moons and rings, comparison "The Chapter, 'The Origin and Evolution of Saturn, with the other gas giant planet, Jupiter, analogies to with Exoplanet Perspective', is to be published by the extrasolar giant planets, as well as pertinent Cambridge University Press as part of a multi- theoretical models. volume work edited by Kevin Baines, Michael Flasar, Norbert Krupp, and Thomas Stallard, entitled 2.1 Introduction “Saturn in the 21st Century” (‘the Volume’) Saturn, though about one-third the mass of Jupiter, is © in the Chapter, Sushil K. Atreya, Aurélien Crida, the largest planetary system in the solar system, Tristan Guillot, Jonathan I. Lunine, Nikku considering the vast reach of its rings and dozens of Madhusudhan, and Olivier Mousis © in the Volume, known moons. Thus, Saturn is key to understanding Cambridge University Press the origin and evolution of the solar system itself. Models, observations, comparison with Jupiter, the NB: The copy of the Chapter, as displayed on this other gas giant planet, and analogies with extrasolar website, is a draft, pre-publication copy only. The giant planets have begun to give a sense of how final, published version of the Chapter will be Saturn, in particular, and the giant planets in general, available to purchase through Cambridge University originated and evolved. Press and other standard distribution channels as part of the wider, edited Volume, once published. Two distinct mechanisms of giant planet formation This draft copy is made available for personal use have been proposed in the literature: (1) disk only and must not be sold or re-distributed." instability (or “grey” instability) and (2) nucleated instability (or core accretion). The latter goes back to Abstract papers by Hayashi (1981) and his colleagues (e.g. Mizuno, 1980), and requires the accretion of a solid Saturn formed beyond the snow line in the primordial body (rock/metal, ice, and possibly, refractory solar nebula that made it possible for it to accrete a organics) up to a critical mass threshold at which rapid large mass. Disk instability and core accretion models accretion of gas becomes inevitable—typically 10 have been proposed for Saturn’s formation, but core times the mass of the Earth (see Armitage, 2010, for a accretion is favored on the basis of its volatile discussion). The former had its origin in the 1970’s abundances, internal structure, hydrodynamic models, (see Cameron, 1979) for hot, massive disks, but it was chemical characteristics of protoplanetary disk, etc. determined later (Boss, 2000; Mayer et al., 2002) that The observed frequency, properties and models of the instabilities required to break up a portion of a exoplanets provide additional supporting evidence for gaseous disk into clumps are a feature of cold, massive core accretion. The heavy elements with mass greater disks. We focus on each of these contrasting models in than 4He make up the core of Saturn, but are presently turn, and then discuss the observational indicators in poorly constrained, except for carbon. The C/H ratio is our own and extrasolar planetary systems that might super-solar, and twice that in Jupiter. The enrichment distinguish between the two models. of carbon and other heavy elements in Saturn and Jupiter requires special delivery mechanisms for The disk instability model is based on numerical volatiles to these planets. In this chapter we will simulations showing that massive, relatively cold review our current understanding of the origin and disks will spontaneously fragment due to a evolution of Saturn and its atmosphere, using a multi- gravitational instability, leading to multiple discrete, self-gravitating masses. In computer simulations of enrichment of heavy elements as well. The model’s the process these features seem somewhat ill-defined, Achilles heel is the time required to build the heavy and it is not possible to track the subsequent element core to the point where rapid gas accretion condensation of these features in the same occurs—millions of years or more. The onset of rapid hydrodynamical simulation that tracks the onset of the gaseous accretion, by which point further growth may instability itself. Nonetheless, basic disk physics be rapid, depends not only on the core accretion rate dictates that such fragmentation will occur for a but also, through the critical core mass (roughly 10 ME, sufficiently massive or cold disk (Armitage, 2010), where ME is an Earth mass) needed to trigger rapid gas and that the timescale for the fragmentation once the accretion, the envelope opacity and hence metallicity. instability does occur is extremely short—hundreds to Furthermore, the core accretion rate itself is a sensitive thousands of years. function of what one assumes about the planetesimal size distribution and surface density in the disk. Once formed, the fragments (assuming they continue to contract to form giant planets) are usually A plausible timescale for the formation of Saturn sufficiently numerous that the aggregate planetary must be consistent with the lifetime of gas in disks, system is dynamically unstable. The planets will but may also be constrained by the 3-5 million year gravitationally interact, scattering some out of the estimate of the formation duration of Iapetus from its system and leaving the others in a variety of possible geophysical shape and thermal history (Castillo- orbits. The evidence from microlensing of a Rogez et al., 2009). Earliest models had lengthy substantial population of free-floating Jupiter-mass formation times (e.g. 8 Myr; Pollack et al., 1996) but objects (Sumi et al., 2011) − not associated with a more recent models can make Saturn in a few million parent star − constitutes one argument in favor of the years by appropriate selection of nebular parameters importance of this formation mechanism. such as grain distribution and opacity (Dodson- Robinson et al., 2008). On the other hand, it is not evident how giant planets formed by the disk instability mechanism acquire The overall history of the solar system and presence significant amounts of heavy elements over and of a substantial terrestrial planet system inward of above their parent star’s abundances. It has been Jupiter and Saturn suggests that the extreme argued that subsequent accretion of planetesimals dynamical scattering suffered after disk instability would generate the increased metallicity, but the protoplanets are formed did not happen in our solar disruption of the disk associated with the system. Furthermore, if the 3-5 million year estimate gravitational instability might have removed the raw of the interval between the formation of the first material for large amounts of planetesimals—the solids and the formation of Iapetus (Castillo-Rogez et materials going into numerous giant planets that are al., 2009) is correct, the disk instability − if it then kicked out of the system. A subsequent phase of occurred − would have produced Saturn much too disk building or direct accretion of planetesimals soon after (or even before), the first solids in the solar from the surrounding molecular cloud may have to be system condensed out. There is sufficient evidence invoked. And this begs the question of core formation that the first solids, millimeter size chondrules and − giant planets formed in this way may have super- calcium aluminum inclusions (CAI’s) in chondrites, solar metallicities but lack a heavy element core date back to 4.5682 Gyr (Amelin et al., 2010), which unless (as seems unlikely) very large (Earth-sized) provides clear evidence that submicron size planets are consumed by these objects. interstellar grains were sticking and accumulating to form solids at the very beginning of the solar system. The core accretion model, in contrast, begins by building a heavy element core through planetesimal Measurement by the Juno mission of the water and embryo accretion in the gaseous disk (embryo is abundance below the meteorology layer in Jupiter, tied usually reserved for lunar-sized bodies and upward). At to the abundances of other major elements measured some point, the gravitational attraction of the large core by the Galileo probe, will also provide an indication of leads to an enhanced accretion of gas, so much so that how much planetesimal material was accreted (Helled gas accretion quickly dominates in a runaway process and Lunine, 2014), and to some extent, the nature of and the object gains largely nebular-composition gas the carrier species (e.g., Mousis, 2012). Although it is until its mass is large enough to create a gap in the disk possible to enrich the envelopes of the giant planets and slow accretion. Such a model produces, by even in the disk instability model by adding definition, a heavy element core, and through co- planetesimals much later, the presence of both a accretion of gas and planetesimals an envelope substantial (10 ME) core and envelope enrichment of heavy elements would strongly militate in favor of the 2.2 Observational constraints core accretion model. Saturn’s core mass may be measured by Cassini, but an inventory of the envelope The models of Saturn’s formation and evolution are enrichment of heavy elements and measurement of the constrained
Details
-
File Typepdf
-
Upload Time-
-
Content LanguagesEnglish
-
Upload UserAnonymous/Not logged-in
-
File Pages36 Page
-
File Size-