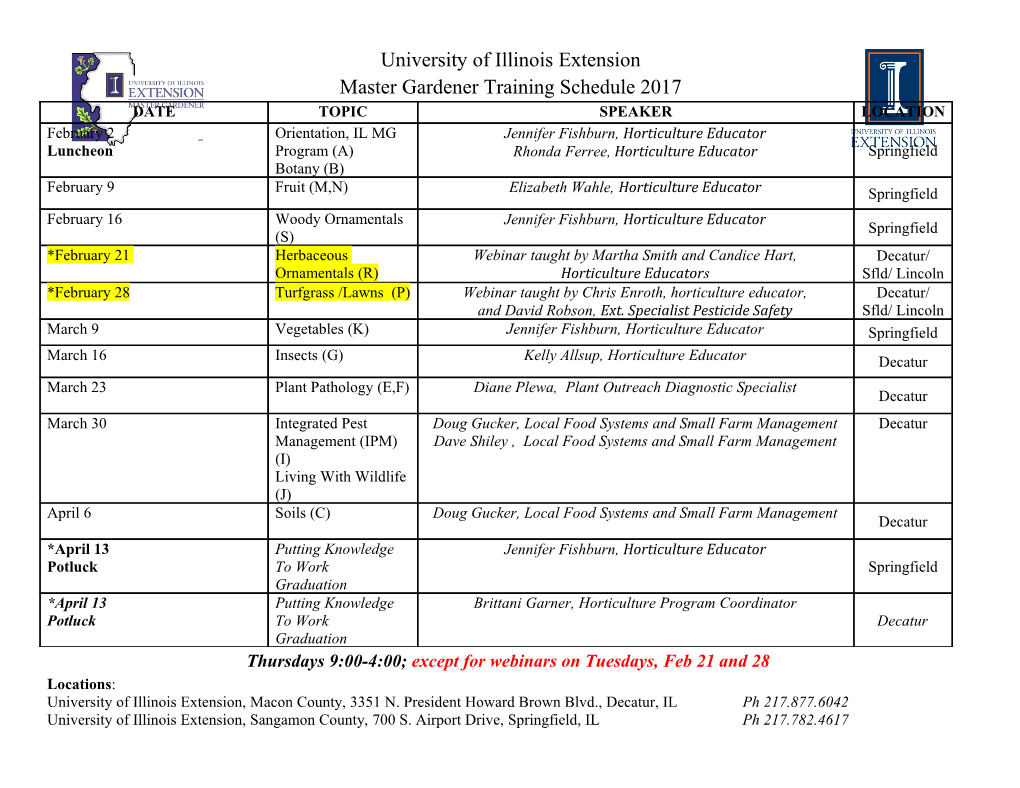
Earth and Planetary Science Letters 308 (2011) 410–416 Contents lists available at ScienceDirect Earth and Planetary Science Letters journal homepage: www.elsevier.com/locate/epsl Size of the group IVA iron meteorite core: Constraints from the age and composition of Muonionalusta Nicholas A. Moskovitz a,⁎, Richard J. Walker b a Carnegie Institution of Washington, Department of Terrestrial Magnetism, 5241 Broad Branch Road, Washington, DC 20015, USA b University of Maryland, Department of Geology, College Park, MD 20742, USA article info abstract Article history: The group IVA fractionally crystallized iron meteorites display a diverse range of metallographic cooling rates. Received 10 December 2010 These have been attributed to their formation in a metallic core, approximately 150 km in radius, that cooled Received in revised form 6 June 2011 to crystallization in the absence of any appreciable insulating mantle. Here we build upon this formation Accepted 11 June 2011 model by incorporating several new constraints. These include (i) a recent U–Pb radiometric closure age of Available online 30 June 2011 b2.5 Myr after solar system formation for the group IVA iron Muonionalusta, (ii) new measurements and Editor: T. Spohn modeling of highly siderophile element compositions for a suite of IVAs, and (iii) consideration of the thermal effects of heating by the decay of the short-lived radionuclide 60Fe. Our model for the thermal evolution of the Keywords: IVA core suggests that it was approximately 50–110 km in radius after being collisionally exposed. This range iron meteorites is due to uncertainties in the initial abundance of live 60Fe incorporated into the IVA core. Our models define a planetary differentiation relationship between cooling rate and closure age, which is used to make several predictions that can be early Solar System tested with future measurements. In general, our results show that diverse cooling rates and early U–Pb closure ages can only coexist on mantle-free bodies and that energy released by the decay of 60Fe reduces the core size necessary to produce diverse metallographic cooling rates. The influence of 60Fe on cooling rates has largely been neglected in previous core formation models; accounting for this heat source can affect size estimates for other iron meteorite cores that cooled to crystallization in the presence of live 60Fe. Candidates for such a scenario of early, mantle-free formation include the iron IIAB, IIIAB and IVB groups. © 2011 Elsevier B.V. All rights reserved. 1. Introduction 2006; Wasson and Richardson, 2001; Willis and Wasson, 1978; Yang et al., 2008). First, they display the widest range of metallographic Amongst the oldest melted rocks in the solar system, fractionally cooling rates (100–6600 K/Myr) of any iron meteorite group (Yang crystallized iron meteorites provide insight on the early stages of et al., 2008). These rates were recorded during the formation of the planet formation. These meteorites are interpreted as fragments Widmanstätten pattern (WP, a structure of interleaved bands of of cores from planetesimals that melted and subsequently differen- kamacite and taenite) as the core cooled from 1000 to 700 K. In tiated due to heating by the decay of short-lived radioactive isotopes addition, the diameters of cloudy zone (CZ) particles at the like 26Al and 60Fe (Goldstein et al., 2009; Mittlefehldt et al., 1998; boundaries of taenite crystals suggest that the IVA cooling rates Moskovitz and Gaidos, 2011). Though these meteorites are highly between 600 and 500 K varied by a factor of fifteen (Yang et al., 2007). evolved, differences in their composition and structure provide a basis Second, several IVAs contain silicate inclusions, which have been for classification (Haack and McCoy, 2005). Fourteen well-defined explained through a host of impact and melt evolution scenarios groups of iron meteorites have been identified, most of which are (Haack et al., 1996; McCoy et al., in press; Ruzicka and Hutson, 2006; thought to represent the cores of distinct parent bodies (Goldstein et Ulff-Møller et al., 1995; Wasson et al., 2006). Third, they are al., 2009). While the classification of these groups is generally agreed significantly depleted in moderately volatile siderophile elements upon, details of their formation, such as parent body size, are less relative to chondrites and other iron groups (McCoy et al., in press; certain. Wasson and Richardson, 2001; Yang et al., 2008). Lastly, though not The origin of the group IVA iron meteorites has long been debated exclusive to the IVAs, their trace element abundances are consistent due to several unusual properties (Haack et al., 1996; Rasmussen et with sampling a majority of their parent core's fractional crystalliza- al., 1995; Ruzicka and Hutson, 2006; Scott et al., 1996; Wasson et al., tion sequence (McCoy et al., in press; Ruzicka and Hutson, 2006; Scott et al., 1996; Wasson and Richardson, 2001). One formation scenario that reasonably explains these properties ⁎ Corresponding author. (see Yang et al. (2008) and Ruzicka and Hutson (2006) for overviews E-mail address: [email protected] (N.A. Moskovitz). of other models) suggests that the differentiated IVA parent body was 0012-821X/$ – see front matter © 2011 Elsevier B.V. All rights reserved. doi:10.1016/j.epsl.2011.06.010 N.A. Moskovitz, R.J. Walker / Earth and Planetary Science Letters 308 (2011) 410–416 411 originally ~1000 km in diameter prior to a hit-and-run collision with a al. (in press).Forthesestartingparameters,theReandOs comparably massive proto-planet (Asphaug et al., 2006; Yang et al., concentrations of Muonionalusta are attained after ~60% fractional 2007). This collision released a string of metal-rich fragments that crystallization, assuming that it has a composition consistent with an eventually cooled to crystallization without the insulating effects of an equilibrium solid. Appropriate concentrations are attained after 50% overlying silicate mantle. In this scenario, the isolated core that would fractional crystallization if Muonionalusta has a composition consis- eventually be disrupted to produce the IVA meteorites must have tent with that of the evolving IVA liquid. Mixtures of solid and liquid been ~300 km in diameter to reproduce the wide range of metallo- compositions are achieved by intermediate extents of fractional graphic cooling rates. A lack of insulating mantle is necessary to crystallization. This result is generally consistent with the model of achieve rapid cooling (N1000 K/Myr) near the surface and the large Wasson and Richardson (2001), who generated a composition similar size ensures that the center of the body cools slowly (~100 K/Myr). to Muonionalusta after ~40% and ~55% fractional crystallization, using This formation scenario merits revisiting in light of a recent U–Pb Au versus Ir and As versus Ir plots, respectively. It is also consistent age for the IVA Muonionalusta, which indicates system closure (i.e. with Yang et al. (2008) who showed that an iron with 8.4% Ni-content cooling below ~600 K) at 4565.3±0.1 Ma (Blichert-Toft et al., 2010). like Muonionalusta would crystallize after ~60% solidification of a core This is the earliest measured age for any fractionally crystallized iron. with initial 3 wt.% S. It falls only ~1 Myr after the basaltic angrite Asuka 881394, the oldest For an inwardly crystallizing core, as expected for the IVA parent differentiated rock in the Solar System (Wadhwa et al., 2009), and is (Haack and Scott, 1992; Ruzicka and Hutson, 2006; Yang et al., 2008), less than 2.5 Myr after the formation of CAIs (calcium–aluminum-rich these data suggest that Muonionalusta formed between approximately inclusions), generally considered to be the first solids to condense 80–70% of the parent body radius, with 70% representing the best fitto from the solar nebula. The aim of this paper is build upon the IVA our data (Fig. 1). This sub-surface origin is consistent with Muoniona- formation model first presented in Yang et al. (2007) by taking into lusta's Ni abundance (an indicator of cooling rate), which is intermediate account newly available constraints on the evolution of the IVA parent to other IVAs (Blichert-Toft et al., 2010; Yang et al., 2008). core. In Section 2 we present a fractional crystallization model to provide context for the origin of Muonionalusta relative to other IVAs. 3. Thermal conduction model In Section 3 we outline a thermal conduction model that includes heating by the decay of 60Fe. This thermal model and the result of the Because of the need for rapid cooling, our model, like that of Yang fractional crystallization calculation are used to constrain the size of et al. (2007), begins with a body without any insulating silicate the IVA core and make predictions for the closure ages of other IVAs mantle. This model is based on the 1D thermal conduction equation (Section 4). In Section 5 we discuss the sensitivity of our results to (Moskovitz and Gaidos, 2011) and assumes a metallic sphere with the various assumptions inherent to the thermal model. In Section 6 we following properties: density 7500 kg/m3, thermal conductivity summarize and discuss the broader implications of this work. 50 W/m/K, specific heat 400 J/kg/K, initial uniform temperature of 1750 K, and a fixed boundary temperature of 200 K. These material 2. Model of IVA fractional crystallization properties are within 20% of those used in other thermal models for differentiated bodies and are generally applicable to iron alloys To estimate the extent of fractional crystallization required to (Ghosh and McSween, 1998, 1999; Hevey and Sanders, 2006; produce Muonionalusta we model the IVA system assuming 3% and Moskovitz and Gaidos, 2011; Yang et al., 2007). The initial and 0.1% initial S and P, respectively, using an approach similar to that of boundary temperatures match those used by Yang et al.
Details
-
File Typepdf
-
Upload Time-
-
Content LanguagesEnglish
-
Upload UserAnonymous/Not logged-in
-
File Pages7 Page
-
File Size-