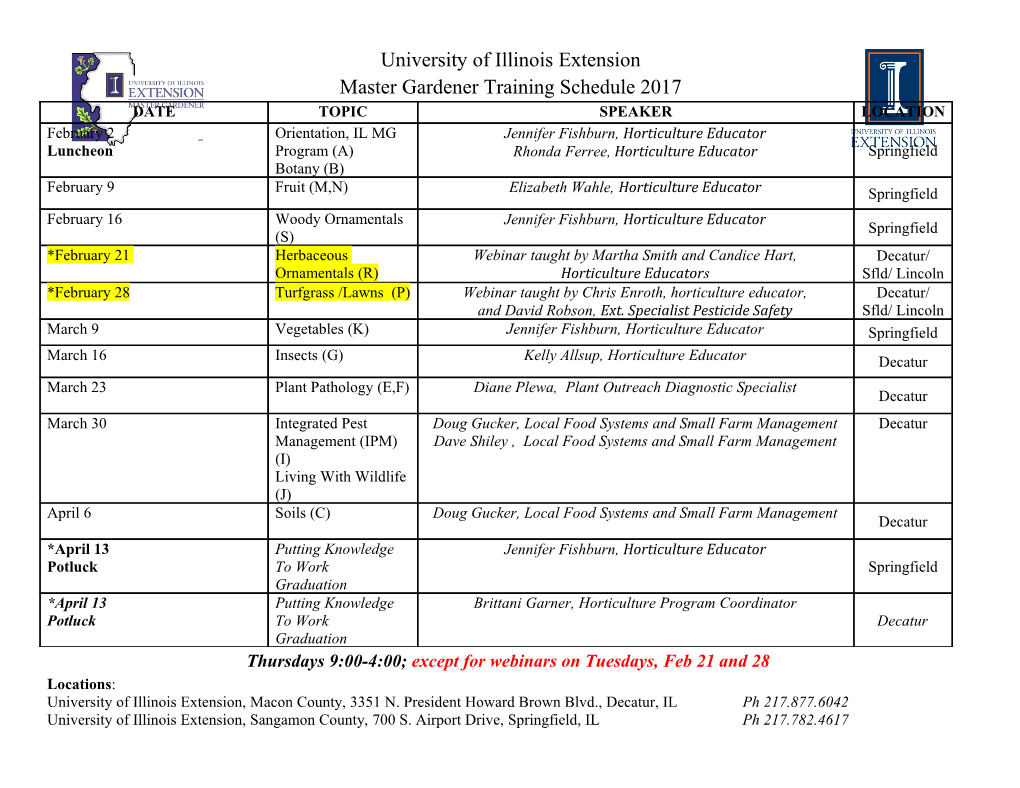
Mercury: Current and Future Science 2018 (LPI Contrib. No. 2047) 6098.pdf TRUE POLAR WANDER OF MERCURY. J. T. Keane1 and I. Matsuyama2; 1California Institute of Technology, Pasadena, CA 91125, USA ([email protected]); 2Lunar and Planetary Laboratory, University of Arizona, Tucson, AZ 85721, USA. Introduction: The spin of a planet is not constant to the Sun and subject to stronger tidal and rotational with time. Planetary spins evolve on a variety of time- forces. However, even with this corrected dynamical scales due to a variety of internal and external forces. oblateness, the required orbital configuration appears One process for changing the spin of a planet is true po- unreasonable−requiring semimajor axes <0.1 AU [5]. lar wander (TPW). TPW is the reorientation of the bulk An alternative explanation may be that a large fraction planet with respect to inertial space due to the redistri- of Mercury’s figure is a “thermal” figure, set Mercury’s bution of mass on or within the planet. The redistribu- close proximity to the Sun and its unique spin-orbit res- tion of mass alters the planet’s moments of inertia onance [7-8] (which are related to the planet’s spherical harmonic de- True Polar Wander of Mercury: While Mercury’s gree/order-2 gravity field). This process has been meas- impact basins and volcanic provinces cannot explain ured on the Earth, and inferred for a variety of solar sys- Mercury’s anomalous figure, they still have an im- tem bodies [1]. TPW can have significant consequences portant effect on planet’s moments of inertia and orien- for the climate, tectonics, and geophysics of a planet. tation. Removing all of Mercury’s mass anomalies re- In this work, we investigate the possibility of TPW veals a figure misaligned with the present-day figure of of Mercury using the high quality geophysical dataset Mercury by ~20°. The Caloris basin, Sobkou basin, and returned by the MESSENGER mission [e.g. 2-4]. The northern volcanic rise each resulted in 5-10° of reorien- last investigation of true polar wander of Mercury used tation (true polar wander). Figure 1 shows a preliminary pre-MESSNEGER data [5]. We find that Mercury’s TPW path of Mercury, in response to the formation of large impact basins and volcanic provinces likely reori- these large impact basins and volcanic provinces ented the planet by tens of degrees. Figure 1: Prelim- Methodology: We have developed a technique for inary true polar isolating the contribution of geologic features to the mo- wander chronol- ments of inertia of planetary bodies [6]. Since most ogy of Mercury. Each point is an planetary-scale features (e.g. impact basins) are axisym- inferred paleo- metric, we model the gravity fields of Mercury’s large pole based on the gravity anomalies using a set of concentric spherical gravity anomaly associated with caps. Spherical caps are advantageous both for their Mercury’s impact simplicity and because their degree-2 gravity field can basins and vol- be directly determined by fitting their higher-order grav- canic provinces. ity field. We fit for the mass anomalies of around a dozen mass anomalies on Mercury, including Caloris, This large Sobkou, the northern rise, and several unidentified grav- magnitude of in- ity anomalies in the southern hemisphere. Despite the ferred TPW has a variety of important consequences for low resolution of the global gravity field of Mercury, the the geology of Mercury. TPW may help explain the na- field is of sufficient quality to do this analysis as we are ture/orientation of Mercury’s fault population. TPW of primarily interested in long-wavelength structures. Mercury likely affected the long-term stability of polar Mercury’s Anomalous Figure: Mercury, like the volatiles [9]. The reorientation of Mercury may also be Moon, possesses a significantly larger degree-2 gravity recorded in Mercury’s paleomagnetic history [10]. field than expected by hydrostatic equilibrium and Mer- References: [1] Matsuyama, I., Nimmo, F., Mi- cury’s present orbit and rotation state. Mercury’s dy- trovica, J. X. (2014) Annu. Rev. Earth & Planet. Sci., -6 42, 605. [2] Smith, D. E. et al. (2012) Science, 336, 214. namical oblateness is J2=50×10 (unnormalized spheri- cal harmonics), while the predicted, hydrostatic dynam- [3] Mazarico, E. et al. (2014) JGR: Planets, 119, 2417. hydrostatic -6 [4] Perry, M. E. et al. (2015) GRL, 42, 6951. [5] Matsu- ical oblateness is only J2 =1×10 . Removing the contribution of impact basins and volcanic provinces yama, I. & Nimmo, F (2009) JGR, 114, E01010. [6] cor- Keane, J. T. & Matsuyama, I. (2014) GRL, 41, 6610. [7] only decreases the dynamical oblateness slightly: J2 rected=41×10-6. There are a few possible explanations for Phillips, R. J. et al. (2014) LPSC, 45, 2634. [8] Tosi, N this excess deformation (sometimes referred to as a et al. (2015) GRL, 42, 7237. [9] Siegler, M. A. et al. “fossil” or “remnant” figure). One explanation is to as- (2016) Nature, 531, 480. [10] Oliveria, J. S. & Hood, L. sume that this figure formed when Mercury was closer L. (2018) Mercury 2018. .
Details
-
File Typepdf
-
Upload Time-
-
Content LanguagesEnglish
-
Upload UserAnonymous/Not logged-in
-
File Pages1 Page
-
File Size-