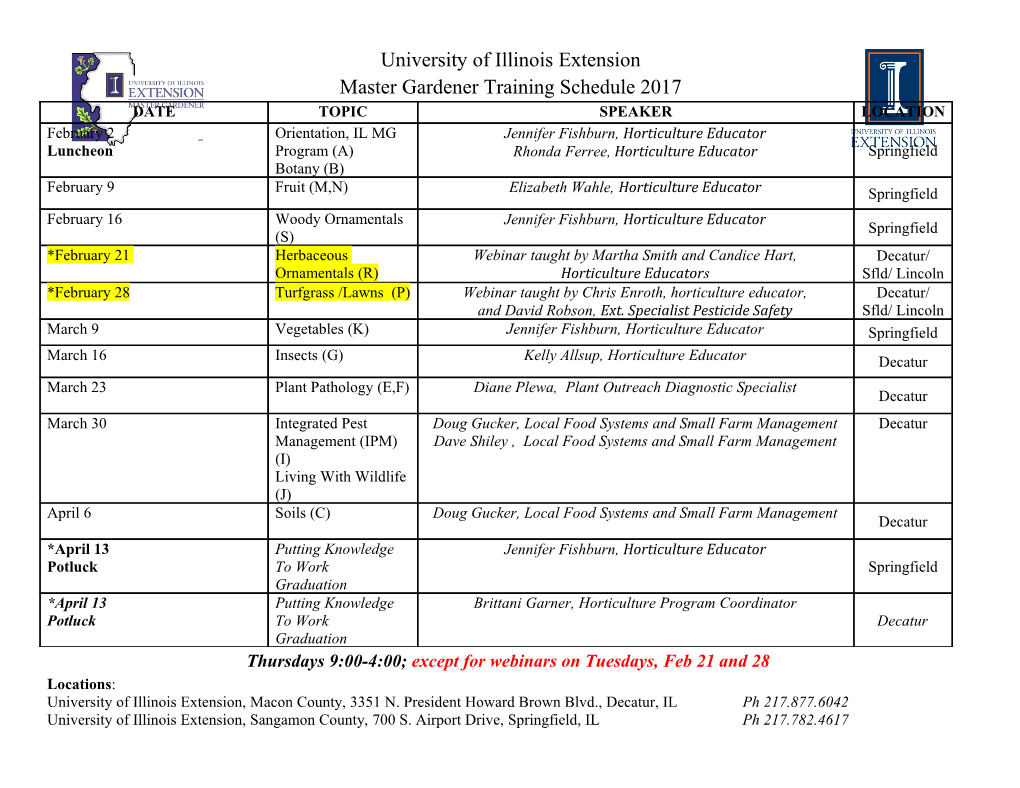
INTRODUCTION Prokaryotes are essential components of the marine ecosystem. They account for the bulk of the biomass and have essential roles in various biogeochemical processes such as carbon, sulfur and nitrogen cycling (Azam et al. 1983, Fenchel 1988). Determining the specific prokaryotic composition of the marine microbial community has proven to be difficult due to the inability of many of these microorganisms to be cultured. Fortunately with the advent of advanced molecular techniques, such as those, which utilize ribosomal RNA gene sequences, the detection, identification and enumeration of individual microbial cells without cultivation, is now possible (Amann et al. 1995). Historically, microbial identification required isolation of pure culture followed by testing for physiological and biochemical traits. Culturable counting techniques such as plate count or most-probable-number (MPN) techniques have been used for quantification of active microbes in environmental samples (Amann et al. 1995). However, direct microscopic counts exceed culturable cell counts by several orders of magnitude (Amann et al. 1995, Fuhrman et al. 1994). This is due to microorganisms that currently are nonculturable as well as culturable cells that exist in a viable-but-non- culturable state (Whitesides and Oliver 1996). The cultured species of the domains of Bacteria (eubacteria) and Archaea (archaebacteria) are known to represent only a small fraction of the actual prokaryotic community (Ferguson et al. 1984, Jannasch and Jones 1959). The domain Bacteria is a highly diverse collection of prokaryotes with numerous culturable species. Whereas the domain Archaea is composed of the kingdom Crenarchaeota, whose cultured members include extreme thermophiles, and the kingdom Euryarchaeota, whose cultured members include methanogens, the extreme halophiles, and sulfur reducing thermophiles (Prescott et al. 1999). Recently, a third kingdom, the Korarchaeota, has been proposed based on sequences collected from hot spring environments and are yet to be cultured (Barns et al. 1996, Pace 1997). The analysis of gene sequences of the small subunit of ribosomal RNA as phylogenetic markers has helped characterize the marine microbial community (Amann et al. 1995, Schmidt et al. 1991). By using PCR amplification, retrieved sequences can be compared and the identity of prokaryotes in natural samples can be determined, regardless of culturablity. One of the first uses of this molecular technique was the analysis of the composition of marine picoplankton (planktonic organisms with an average diameter of 0.2-2.0µm). Studies revealed that most marine prokaryotes were undescribed species that had not been cultivated (Britschgi and Giovannoni 1991, DeLong 1992, Fuhrman et al.1992, Mullins et al. 1995). Recent phylogenic studies have shown that uncultured crenarchaeotes (referred to as group I archaea) have been discovered in marine plankton (DeLong 1992, DeLong et al. 1994, Fuhrman and Davis 1993, Fuhrman and Davis 1997, Massana et al. 1997), marine and freshwater sediments (Kato et al. 1997, MacGregor et al. 1997, McInerney et al. 1995, Ravenschlag et al. 2000), freshwater plankton (Jurgens et al. 2000), deep sea hydrothermal vent fluids (Huber et al. 2002), and marine animals (McInerney et al. 1995). Uncultured euryarchaeotes referred to as group II archaea have been found in marine plankton (DeLong 1992, DeLong et al. 1994, Fuhrman and Davis 1993,1997, Massana et al. 1997), freshwater plankton (Jurgens et al. 2000), marine sediments (Moyer et al. 1998), deep sea hydrothermal vent fluids (Huber et al. 2002), and the digestive tracts of fish (Van der Maarel et al. 1998). Marine archaea are believed to be abundant and could account for up 2 to 30% of the total picoplankton in both polar and temperate coastal waters and up to 60% in both mid and pelagic waters (DeLong et al. 1994, Fuhrman and Davis 1997, Massana et al. 1997, 2000). While marine microbial diversity can be studied by PCR-based phylogenic analysis, the actual community structure cannot be deduced due to potential bias from amplification of certain templates, potential sample contamination, variability in cloning efficiencies for different rRNA gene fragments, and selective priming for certain sequences (Polz & Cavanaugh 1998). By using quantification methods such as fluorescence in situ hybridization (FISH), the identity and abundance of the natural community can be determined (Amann et al. 1995). Fluorescence in situ hybridization is a molecular technique that uses fluorescent probes that specifically target rRNA gene sequences of whole cells in situ, which allows for the identification and enumeration of nonculturable microorganisms in their natural environment (Amann et al. 1995, Delong et al. 1999). In this technique fluorescently labeled rRNA-targeted oligonucleotide probes penetrate the microbial cell wall and bind to the complimentary targeted rRNA sequence and are then detected by epifluorescence microscopy (Amann et al. 1989, DeLong et al. 1989). The distribution, quantification and phylogenetic characters of the marine microbial community have been characterized in deep-sea waters using rRNA molecular analysis (Amann et al. 1995, Britschgi and Giovannoni 1991, DeLong 1992, DeLong et al. 1999, Massana et al. 1996, Massana et al. 1999, Murray et al. 1998, Ward et al. 1992), yet there are few reports of these techniques being applied to the estuarine environment. One report of the archaeaplankton of the Columbia River estuary has indicated that 3 archaea are present in the estuarine environment yet do not form a native estuarine community. This is in contrast with the bacterial community, which seems to form a native estuarine community (Crump and Baross 2000). Estuaries are characterized as that portion of the earth’s coastal zone where there is interaction of ocean water, fresh water, land, and atmosphere. The species composition of the estuarine community is a function of various environmental factors including salinity, turbidity, nutrients, turbulence, and depth. Estuaries tend to have high levels of nutrients many of which are recycled through the activities of microorganisms, and thus are critical in controlling the function and structure of estuarine ecosystems (Day et al. 1989). Because estuaries act as a natural sink for nutrients it is essential to identify those microorganisms that are responsible for the nutrient recycling. The Cape Fear River estuary drains the largest and most industrialized watershed in North Carolina, and is home to 27% of the state's population (Mallin et al. 2000). Freshwater from the Cape Fear River flows into the Cape Fear estuary, which is characterized as having a salinity gradient from fresh to salt water and is subject to a tidal influence from the Atlantic Ocean. Like many estuaries, it functions as a nursery for marine animals, a habitat for numerous plants and animals species, a storage basin for nutrients and as a buffer zone from storm impact. The estuarine environment is highly susceptible to chemical runoff and other forms of air and water pollution (Day et al. 1989). Therefore to further understand this dynamic environment, characterization of estuarine microorganisms is needed. The objectives of this study were to quantify the microorganisms of the small eukaryotic (5.0-0.22µm), bacterial and archaeal community of the Cape Fear River 4 estuary, by using fluorescence in situ hybridization (FISH). The archaeal community was further quantified by group-specific analysis. By using FISH the spatial and temporal composition of the planktonic microbial community of the Cape Fear River estuary was analyzed. This study represents the first attempt to detect and quantify marine Archaea in the Cape Fear River estuary. MATERIALS AND METHODS Site Description The Cape Fear River estuary is a coastal plain estuary joined by three major tributaries, The Cape Fear, the Black and the Northeast Cape Fear rivers, in southeastern North Carolina (Figure 1). The system drains numerous swamp forests, which add organic color and acidic tannins to the mainstream. The estuary is influenced by a diurnal tide, which extends upstream northeast from the Atlantic Ocean. It is the most heavily industrialized watershed within North Carolina with approximately 1.5 million people living within its basin (Mallin et al. 2002). Sample collection, processing, and storage Water samples were collected monthly during out-going tides at surface and depth (mean 11m) for one year, at three sites along the Cape Fear River estuary. Samples were taken in conjunction with the Lower Cape Fear River Monitoring Project. Numbered sites (Figure 1) were as follows: (1) Channel Marker 18, located near the mouth of the Cape Fear River estuary with a marine salinity range; (2) Channel Marker 42, located near Keg Island, mid-estuary with a mesohaline salinity range; and (3) Navassa, located 5 3 • 2 • 3, 4 • 1 Figure 1. Map of sites sampled from September 2001 to August 2002. 6 near the head of the estuary, with a oligohaline salinity range. Samples were collected in sterile glass bottles and stored on ice until processed in the laboratory (within 6 hours). Physical parameters were measured with each sample using a Yellow Springs Instrument 6920 (YSI; Yellow Springs, Ohio) Multiparameter Water Quality Probes and stored on a YSI 610D datalogger. Physical parameters included water temperature, salinity, pH, turbidity and dissolved oxygen. Standard Plate Count Standard plate count was performed to compare
Details
-
File Typepdf
-
Upload Time-
-
Content LanguagesEnglish
-
Upload UserAnonymous/Not logged-in
-
File Pages28 Page
-
File Size-