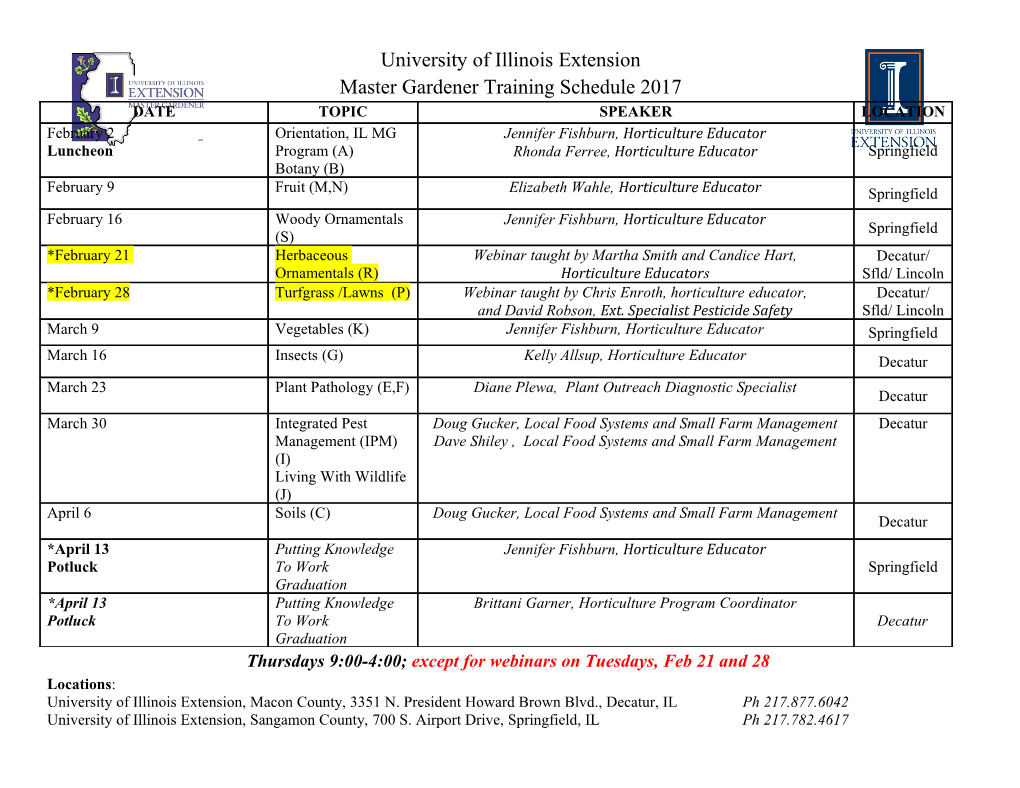
week ending PRL 101, 114503 (2008) PHYSICAL REVIEW LETTERS 12 SEPTEMBER 2008 Nanofluidics in the Debye Layer at Hydrophilic and Hydrophobic Surfaces C. I. Bouzigues,1 P. Tabeling,1,* and L. Bocquet2,3 1Laboratoire de Microfluidique, MEMS et Nanostructures, UMR CNRS Gulliver-ESPCI 10, rue Vauquelin 75005 Paris, France 2LPMCN; Universite´ de Lyon; Universite´ Lyon 1 and CNRS, UMR 5586, F-69622 Villeurbanne, France 3Physics Department, Technical University Munich, 85748 Garching, Germany (Received 15 May 2008; published 9 September 2008) By using evanescent waves, we study equilibrium and dynamical properties of liquid-solid interfaces in the Debye layer for hydrophilic and hydrophobic surfaces. We measure velocity profiles and nanotracer concentration and diffusion profiles between 20 and 300 nm from the walls in pressure-driven and electro- osmotic flows. We extract electrostatic and zeta potentials and determine hydrodynamic slip lengths with 10 nm accuracy. The spectacular amplification of the zeta potential resulting from hydrodynamic slippage allows us to clarify for the first time the dynamic origin of the zeta potential. DOI: 10.1103/PhysRevLett.101.114503 PACS numbers: 47.61.Àk, 47.80.Cb The development of microfluidics and nanofluidics has enlightening it could be, has not received an experimental raised new challenges to drive and manipulate flows in ever confirmation yet. In this work, we use a total internal more tiny channels [1,2]. One key question concerns the reflection fluorescence (TIRF) technique [19,20] to explore nature of the boundary condition of fluids at solid surfaces. simultaneously liquid dynamics and electrostatic poten- Recent studies have demonstrated that hydrophobic sur- tials between 20 and 300 nm from both hydrophilic and faces develop hydrodynamic slippage at solid surfaces, as hydrophobic surfaces. The combination of evanescent quantified by the so-called slip length b [3–5]. Slip lengths waves to illuminate the flow, as well as a specific tracking on the order of a few tens of nanometers are typically technique and nanometric particles to seed the fluid, allows reported for clean hydrophobic surfaces [6–9]. However us to bypass the optical limitations inherent to classical encouraging, this is insufficient to obtain a substantial particle velocimetry (PIV) techniques [8,21]. As a result, decrease of the viscous friction experienced by pressure- we bring the first experimental evidence for a slip-induced driven flows in channels of micrometric size or larger. amplification effect, thus demonstrating that the zeta po- Increasing slip lengths by orders of magnitude is feasible tential is not a purely electrostatic quantity, but also in- by patterning the surfaces [10]; nonetheless, with the cludes a large contribution originating from hydrodynamic present state-of-art technology, these surfaces still remain slip. delicate to exploit. The situation is different for electro- Throughout the experiments, we used 20 nm sulfate con- osmotic flows, i.e., flows induced by the coupling between jugated polystyrene fluorescent particles (Fluospheres, surface charges and externally applied electric fields. In Molecular Probes), seeding ultrapure water (MilliPore, this case, it has been theoretically shown [5,11–13] that 18:2M ), at a 0.02% concentration. The nanoparticle slip lengths in the nanometric range are sufficient to con- (NP) surface potential p was measured by a zetameter siderably amplify the electro-osmotic (EO) speeds. This apparatus. In our experimental conditions, we measured amplification effect is embodied in the surface zeta poten- p ¼5:8 Æ 1:2mV, corresponding to qp ¼ 3:0 Æ 0:6e tial , which enters EO mobility as EO ¼ = (with per NP (assuming a thick electrical layer). the dielectric constant and the fluid viscosity): Microchannels (10  100 m) are made in polydime- Refs. [12,13] predicted that is related to the electrostatic thylsiloxane (PDMS) using standard soft lithography tech- surface potential É0, according to ¼ É0ð1 þ b=DÞ niques [22], and closed by a 170 m thick microscope where D is the nanometric Debye length characterizing glass slide. In the case of electro-osmotic flows, a pair of the width of the electric double layer at the surface [14]. gold electrodes, separated by 2 cm, is deposited onto the This leads accordingly to a ð1 þ b=DÞ slip-induced am- glass surface. Flows are either pressure driven or electro- plification factor of . Under realistic conditions, one may osmotically driven: typical pressure drops are hundreds of obtain a tenfold amplification of the bulk velocity just by Pa, and in the latter, voltages applied across the two increasing the slippage at the wall by a few nanometers. electrodes are 10 V. The maximum speeds in the micro- Such a spectacular phenomenon has recently attracted channel ranged between 20 and 50 m Á sÀ1. Experiments much attention in the context of energy conversion [15]. were performed on a DM-IRM microscope equipped with One may suspect that it plays a role in the development of a 100 NA ¼ 1:46 objective (Leica). Flowing liquid was large zeta potentials consistently observed for hydrophobic illuminated by a laser beam (Sapphire 488, Coherent) with surfaces [16,17]; recently, it was used to interpret a number a continuously tunable incidence angle i (Fig. 1). Tracers of observations [18]. The theoretical prediction, however were imaged in a field of view of 80  80 m under 0031-9007=08=101(11)=114503(4) 114503-1 Ó 2008 The American Physical Society week ending PRL 101, 114503 (2008) PHYSICAL REVIEW LETTERS 12 SEPTEMBER 2008 FIG. 1 (color online). Left: Scheme of the TIRF setup. A laser beam is collimated through a high numerical aperture objective (100 NA ¼ 1:46) with an incidence angle higher than the critical angle for total reflection. Laser is focused in the back focal plane of the objective with an achromatic doublet (focal length 150 mm) and the incidence angle is tunable by a rotating mirror in the optical path. The evanescent wave created within the channel restricts fluorescence to tracers (black dots) in the vicinity of the surface. Right: Typical images obtained by TIRF illumination: the two images are acquired in frame-transfer mode with an exposure/ acquisition time of 40 ms. Scale bar represents 5 m. 50 mW illumination on an electron multiplying CCD by the surface is given by ÉðzÞ¼4kBT= À1 camera (Ixon Andor) with an acquisition rate of 25 Hz in etanh ½ tanh½eÉ0=4kBT expðz=DÞ, where É0 is the frame-transfer mode. When i is larger than the critical surface potential and D the Debye length [14]. Experi- angle of refraction at a glass-water interface, the illumina- mental profiles are fitted using this expression with both tion power within the channel is given by IðzÞ¼ the Debye length and surface energy (qpÉ0=kBT) as free I0 expðz=LeÞ, in which Le is the penetration length. parameters. Predictions for the density profiles are shown This quantity is determined by measuring the aspect ratio in Fig. 2(a), showing good agreement with experiment: we qpÉ0 of the laser waist in the sample plane. Under our experi- obtain accordingly É0 ¼69 Æ 20 mV ( ¼8:1 Æ kBT mental conditions of TIRF, Fig. 1, Le was varied between 0:9) for the hydrophilic surface and É0 ¼65 Æ 19 mV 150 and 400 nm. Given the monodispersity of particle q É ( p 0 ¼7:5 Æ 0:7) for the hydrophobic OTS surface. We emission (we measured 17% standard type deviation in kBT intensity of tracers on the surface), the fluorescence inten- checked that the estimated Debye lengths agree with the sity of a single tracer carries the information on its altitude classical theory for different salt concentrations [14]; see with an accuracy Áz ¼ LÁI=I 17 nm. From the in situ Fig. 2(b). A few remarks are in order. First, the surface measurement of emission intensity of tracers adsorbed on potential for the hydrophilic glass and silanized surface are the surface, one determines I0 and consequently obtains the consistent with reported values obtained for the zeta po- altitudes of each particle traveling close to the surface. tential on similar surfaces [16,24]. Note that the surface We performed experiments on hydrophilic and hydro- phobic surfaces. Hydrophilic surfaces were prepared by A 0.7 B 150 0.6 0.8 washing Pyrex coverslips with detergent, acetone, toluene, 0 0.5 and ethanol. Hydrophobic surfaces were obtained by sila- 0.4 100 C(z)/C 0 0.4 nization of the coverslip with octadecyl-trichloro-silane 0 U/U 0.3 0 100 200 <20 50 (OTS). Contact angles are (glass) and 95 (OTS) 0.2 z (nm) and rms roughnesses measured by atomic force micros- 0.1 Debye length (nm) copy (AFM) are, respectively, 0:33 Æ 0:01 and 0:44 Æ 0 0 50 100 150 200 0123 0:01 nm. z (nm) Salt concentration (10-4 M) We first discuss measurements of the concentration pro- files of NPs as a function of the altitude. The measure- FIG. 2 (color online). (a) Electrostatic interaction energy be- ments, shown in Fig. 2(a), reveal an exponential depletion tween NPs and surface, as deduced from the NP concentration of the density of NPs close to the surface [inset of profile (see inset): open circles ( ), hydrophilic; plain circles d 1:5 10À4 mol LÀ1 Fig. 2(a)], which can be attributed to electrostatic repul- ( ), hydrophobic. Salt concentration is  Á . sion between the NPs and the surfaces [23]. The particle Dashed lines are fits to the PB predictions, providing the Debye length D ¼ 49 Æ 5nmand the energy of interaction of the NPs distribution at thermal equilibrium is given by CðzÞ¼ with the surface: U0 ¼ 8:1 Æ 0:7kBT (hydrophilic) and U0 ¼ C expð q É z =kTÞ C 0 À p ð Þ , where 0 is the bulk concentration 7:5 Æ 0:7kBT (hydrophobic).
Details
-
File Typepdf
-
Upload Time-
-
Content LanguagesEnglish
-
Upload UserAnonymous/Not logged-in
-
File Pages4 Page
-
File Size-