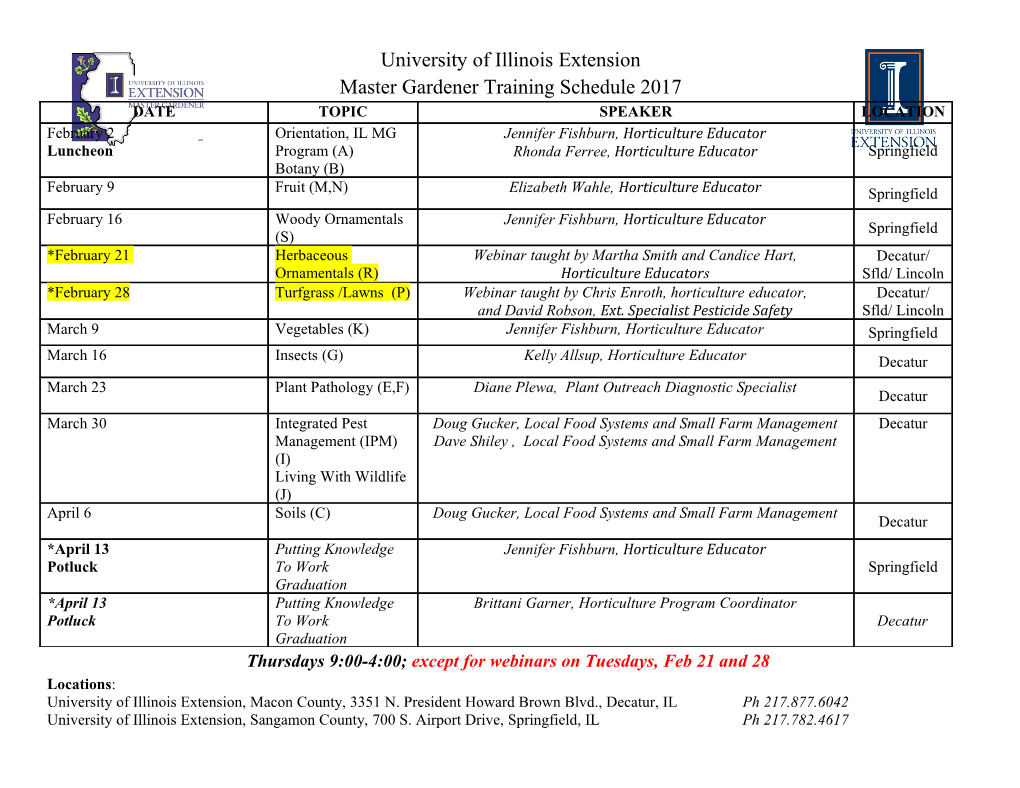
Metamaterial Structures for Wakefield Acceleration and High Power Microwave Generation by Xueying Lu B. S. (Engineering Physics), Tsinghua University (2012) Submitted to the Department of Physics in partial fulfillment of the requirements for the degree of Doctor of Philosophy at the MASSACHUSETTS INSTITUTE OF TECHNOLOGY February 2019 ○c Massachusetts Institute of Technology 2019. All rights reserved. Author................................................................ Department of Physics November 16, 2018 Certified by. Richard J. Temkin Senior Scientist, Department of Physics Thesis Supervisor Accepted by . Nergis Mavalvala Associate Department Head 2 Metamaterial Structures for Wakefield Acceleration and High Power Microwave Generation by Xueying Lu Submitted to the Department of Physics on November 16, 2018, in partial fulfillment of the requirements for the degree of Doctor of Philosophy Abstract This thesis presents the theoretical and experimental investigation of the interac- tion of metamaterial structures with electron beams for two applications: wakefield acceleration and high power microwave generation. Under the topic of wakefield acceleration, on the theoretical side, several meta- material structures have been designed and simulated. The novel phenomenon of reversed Cherenkov radiation has been found to enhance the beam-wave interaction in metamaterials. A metallic wagon wheel metamaterial structure was designed and built for use in an experiment at the Argonne Wakefield Accelerator (AWA) Facility. On the experimental side, this thesis presents the first demonstration of high- power, reversed Cherenkov wakefield radiation by short electron bunches passing through the wagon wheel structure at the AWA. Single 45 nC electron bunches of 65 MeV energy traversing the structure generated up to 25 MW in 2 ns pulses at 11.4 GHz, in excellent agreement with theory. Two bunches of 85 nC with appropriate temporal spacing generated up to 80 MW by coherent wakefield superposition. If this power were applied to a trailing witness bunch in a collinear wakefield accelerator, it would provide an accelerating gradient of 75 MV/m. Under the topic of high power microwave generation, on the theoretical side, an analytical theory has been developed to predict the novel Cherenkov-cyclotron in- teraction in metamaterial-based microwave devices. An S-band metamaterial-loaded waveguide with reverse symmetry has been designed and built to work with the Cherenkov-cyclotron interaction. On the experimental side, this thesis presents the experimental results of the metamaterial-loaded waveguide built at MIT. Power levels to 2.9 MW at 2.4 GHz in full 1 휇s pulses were generated by an electron beam of up to 490 kV of voltage and 84 A of current. Frequency tuning measurements verified that pulses above 1 MW of output power were only seen in the Cherenkov-cyclotron mode. With these results, this thesis demonstrates the unique features of metamaterial structures that are very attractive for high-gradient wakefield accelerators and high power microwave sources. Advantages include the high shunt impedance for intense 3 beam-wave interaction; the simple and rugged structure; and a large parameter space for various optimization goals. Thesis Supervisor: Richard J. Temkin Title: Senior Scientist, Department of Physics 4 Acknowledgments This thesis is the product of the tremendous support and help I was fortunate to receive from many people, to whom I owe a debt of gratitude. I would like to thank my thesis advisor, Dr. Richard Temkin, who has all the great things that an amazing advisor can have: knowledge, insight, patience, willingness to help, and many more. I thank Rick for bringing me to MIT and for making my PhD years enjoyable. It has been my great honor to work in the group. I would like to thank Dr. Michael Shapiro, who also closely advised my research and contributed greatly to the completion of this thesis. A quick talk with Michael would often lead to solutions to some mysteries that troubled me for days or weeks. I would like to thank Dr. Ivan Mastovsky, who always knows how to deal with misbehaving lab equipment. Ivan’s experimental expertise helped me get over all the hurdles during my time in the lab. I would like to thank my colleagues in the group past and present. I am so lucky to get to know and to work with these great people. I thank Dr. Jason Hummelt for showing me how to run the experiment in the West Cell. I thank Dr. Jacob Stephens and Dr. Guy Rosenzweig for their help and good conversations in the lab. I thank Dr. Jiexi Zhang for being a patient climbing buddy. I thank Dr. Alexander Soane for sending postcards from all over the world. I thank Dr. Sudheer Jawla, Julian Picard, Samuel Schaub and Haoran Xu for helpful discussions and comments on my work. I thank my previous officemates Dr. Sergey Arsenyev, Dr. Elizabeth Kowalski and Hannah Hoffmann for making the work environment happy. I would like to thank our collaborators at the Argonne National Laboratory, Dr. John Power, Dr. Manoel Conde, Dr. Jiahang Shao, Dr. Eric Wisniewski, Scott Doran, Charles Whiteford and Dr. Chunguang Jing, for their great help. They made my experience in Argonne both rewarding and fun. I would like to thank the MIT central machine shop, Andrew Gallant and Michael Abruzzese, for making almost all the parts for my experiments and for helping me to improve the mechanical drawings. 5 I would like to thank Dr. Miklos Porkolab and Dr. John Belcher for being on my thesis committee and my oral exam committee. Dr. Earl Marmar also served on my oral exam committee. From them I received comments and suggestions which helped greatly throughout the preparation and the review of this thesis. I would like to thank my undergraduate professors at Tsinghua University, Dr. Wenhui Huang, Dr. Jianfei Hua, Dr. Chuanxiang Tang, Dr. Huaibi Chen and Dr. Yi-Kang Pu, for encouraging me to pursue my graduate study in physics. I would like to thank all my friends, at MIT, in Boston, in the US, and in China, for sharing numerous good memories with me. I am most proud of the friends I have kept for over a decade long: Yan, Jessica Qijie, Yipeng, Zhao, Rui and Zong. During these years, we have grown separately, but we have never grown apart. Last but not least, I would like to thank my parents. My father Chao Lu intro- duced me to classical Chinese literature when I was a kid, which has taught me the great values of kindness, integrity and courage. My mother Xiaoxia Xue provides me with a great example of an independent and strong woman. I thank you for your love and support, and this thesis is dedicated to you. Xueying Lu November 15, 2018 During first snow of the season Cambridge, MA 6 Contents 1 Introduction 21 1.1 Metamaterials . 21 1.1.1 Basic Principles of Metamaterials . 23 1.1.2 Negative Refraction . 26 1.1.3 Reversed Cherenkov Radiation . 28 1.2 Wakefield Acceleration . 29 1.2.1 Motivation for High Gradient Accelerator Research . 30 1.2.2 Plasma Wakefield Acceleration . 32 1.2.3 Structure-Based Wakefield Acceleration . 33 1.2.4 Advantages of Metamaterial Structures for Wakefield Acceleration 37 1.3 High Power Microwave Generation . 38 1.3.1 Metamaterial-Based Microwave Sources . 38 1.3.2 Stimulated Cherenkov Radiation . 39 1.4 Thesis Outline . 42 2 Theory of Metamaterial Structures for Wakefield Acceleration 45 2.1 Motivation . 45 2.2 Theory of Circular Deep Corrugation Structure . 46 2.2.1 Design of the Circular Deep Corrugation Structure . 46 2.2.2 Analytical Theory . 47 2.2.3 Numerical Simulation of a Multi-Cell Structure . 50 7 2.2.4 Benchmarking . 51 2.2.5 Scaling Study . 53 2.2.6 Nominal Design . 60 2.2.7 Conclusions . 61 2.3 Design of Wagon Wheel Structure . 62 2.3.1 Motivation . 62 2.3.2 Unit Cell Design . 63 2.3.3 Double Negative Permittivity and Permeability . 66 2.3.4 Enhanced Beam-Wave Interaction . 67 2.3.5 Output Ports Design . 70 2.4 Theory of Wagon Wheel Metamaterial Structure . 72 2.4.1 Pulse Length . 72 2.4.2 Output Power from a Single Bunch . 75 2.4.3 Output Power from a Bunch Train . 75 2.5 Simulations of Wagon Wheel Metamaterial Structure . 77 2.5.1 Microwave Simulations . 77 2.5.2 Beam Simulations . 79 2.6 Theory of Volumetric 3D Metamaterial Structure . 81 2.6.1 Motivation . 82 2.6.2 Unit Cell Design . 83 2.6.3 Effective Medium Theory with Spatial Dispersion . 87 2.6.4 Wave-Beam Interaction Using Effective Medium Theory . 91 2.6.5 Radiation Pattern in a Volumetric Array of Metamaterial Unit Cells . 95 2.6.6 Application of the Volumetric Metamaterial for Wakefield Ac- celeration . 97 2.6.7 Conclusions . 98 2.7 Theory of Elliptical Deep Corrugation Structure . 100 8 3 Experiment of Metamaterial Structures for Wakefield Acceleration103 3.1 Experimental Facilities . 103 3.2 Structure Fabrication . 110 3.2.1 Full Assembly . 110 3.2.2 Metamaterial Structure Part . 111 3.2.3 Waveguides . 114 3.3 Cold Test . 116 3.3.1 Transmission Measurement . 117 3.3.2 Beadpull Measurement . 117 3.4 Beam Test Results . 124 3.4.1 Single Bunch Experiment . 124 3.4.2 Two-Bunch Experiment . 128 3.5 Conclusions . 137 4 Theory of Metamaterial Structures for High Power Microwave Gen- eration 139 4.1 Review of Previous Metamaterial-Based High Power Microwave Re- search at MIT . 140 4.2 Theory of Cherenkov-Cyclotron Instability in a Metamaterial Waveguide147 4.2.1 Motivation . 147 4.2.2 Metamaterial Loaded Waveguide Mode . 149 4.2.3 Electron Beam Interaction with the Antisymmetric Metamate- rial Waveguide Mode: Cherenkov-Cyclotron Instability .
Details
-
File Typepdf
-
Upload Time-
-
Content LanguagesEnglish
-
Upload UserAnonymous/Not logged-in
-
File Pages218 Page
-
File Size-