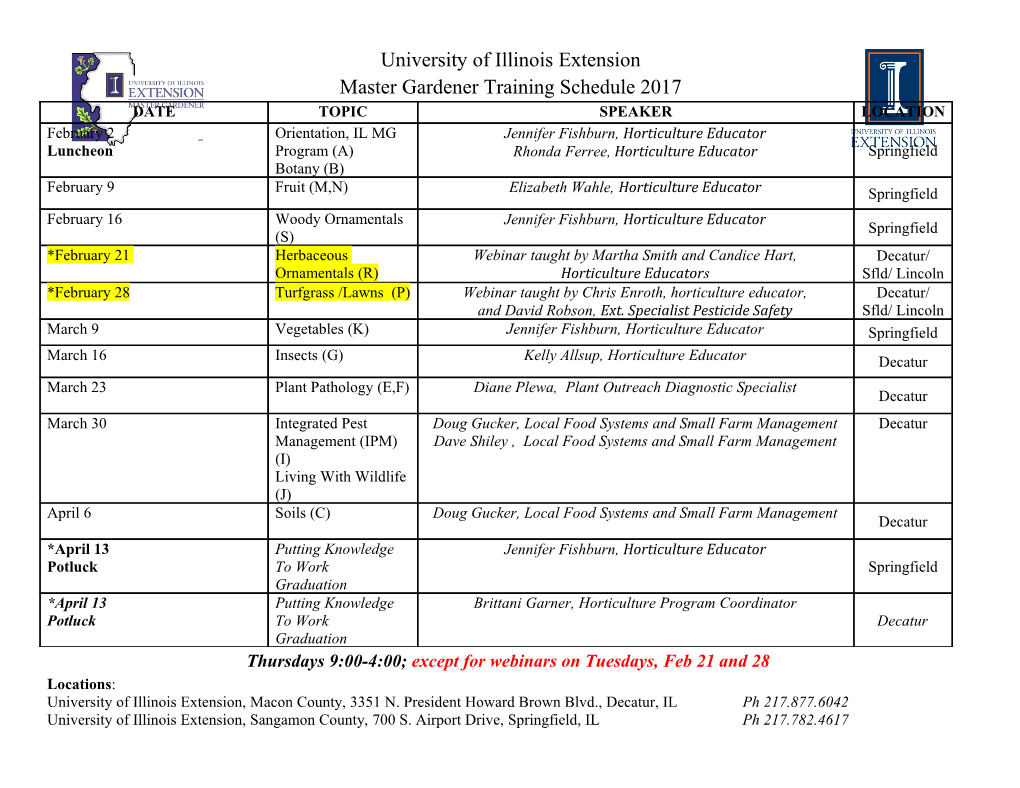
Molecular Aspects of Medicine 31 (2010) 60–74 Contents lists available at ScienceDirect Molecular Aspects of Medicine journal homepage: www.elsevier.com/locate/mam Review The Warburg effect and mitochondrial stability in cancer cells Vladimir Gogvadze, Boris Zhivotovsky, Sten Orrenius * Institute of Environmental Medicine, Division of Toxicology, Karolinska Institutet, Box 210, Stockholm SE-171 77, Sweden article info abstract Article history: The last decade has witnessed a renaissance of Otto Warburg’s fundamental hypothesis, Received 22 June 2009 which he put forward more than 80 years ago, that mitochondrial malfunction and subse- Received in revised form 31 July 2009 quent stimulation of cellular glucose utilization lead to the development of cancer. Since Accepted 2 December 2009 most tumor cells demonstrate a remarkable resistance to drugs that kill non-malignant cells, the question has arisen whether such resistance might be a consequence of the abnormalities in tumor mitochondria predicted by Warburg. The present review discusses Keywords: potential mechanisms underlying the upregulation of glycolysis and silencing of mitochon- The Warburg effect drial activity in cancer cells, and how pharmaceutical intervention in cellular energy Apoptosis Mitochondria metabolism might make tumor cells more susceptible to anti-cancer treatment. Cancer Ó 2009 Elsevier Ltd. All rights reserved. Contents 1. Introduction: The Warburg effect vs. the Pasteur effect – historical background and current views. ....... 61 2. Possible mechanisms of mitochondrial silencing in cancer cells . .......................................... 62 2.1. The Crabtree effect . ...................................................................... 62 2.2. HIF and suppression of mitochondrial activity . ................................................... 62 2.3. Importance of ROS in cancer . ...................................................................... 63 3. Consequences of the glycolytic switch in tumors . ............................................................. 64 4. Mitochondrial stabilization in glycolytic tumor cells............................................................. 65 4.1. The role of Bcl-2 proteins in OMM permeabilization . ................................................... 65 4.2. Hexokinase and OMM stability . ...................................................................... 67 4.3. ANT and OMM stability . ...................................................................... 67 4.4. Akt-mediated OMM stability . ...................................................................... 68 4.5. Contribution of p53 to OMM stability . ................................................... 68 5. Stimulation of cancer cell death via intervention with cellular ATP-generating pathways. .......................... 69 5.1. Suppression of the glycolytic pathway . ................................................... 69 5.2. Shifting metabolism from glycolysis to glucose oxidation ................................................... 70 5.3. Alteration of mitochondrial functions . ................................................... 70 6. Concluding remarks . ................................................................................ 71 Abbreviations: ACL, ATP citrate lyase; ANT, adenine nucleotide translocase; ATRA, All-Trans retinoic acid; 3-BrPA, 3-bromopyruvate; COX, cytochrome oxidase; CsA, cyclosporin A; CyPD, cyclophilin D; DCA, dichloroacetate; HIF-1, hypoxia-inducible factor-1; IMM, inner mitochondrial membrane; LDH, lactate dehydrogenase; mtDNA, mitochondrial DNA; OMM, outer mitochondrial membrane; PDH, pyruvate dehydrogenase; PDK1, pyruvate dehydrogenase kinase 1; PPIase, peptidyl-prolyl cis–trans isomerases; MPT, mitochondrial permeability transition; PI3-K, phosphoinositide 3-kinase; ROS, reactive oxygen species; SCO2, Synthesis of cytochrome c oxidase 2; TIGAR, TP53-Induced glycolysis and apoptosis regulator; a-TOS, a-tocopheryl succinate; VDAC, voltage- dependent anion channel. * Corresponding author. E-mail address: [email protected] (S. Orrenius). 0098-2997/$ - see front matter Ó 2009 Elsevier Ltd. All rights reserved. doi:10.1016/j.mam.2009.12.004 V. Gogvadze et al. / Molecular Aspects of Medicine 31 (2010) 60–74 61 Acknowledgements . ....................................................................... 71 References . .......................................................................................... 71 1. Introduction: The Warburg effect vs. the Pasteur effect – historical background and current views In 1926, Otto Warburg reported that cancer cells produce most of their ATP via glycolysis, also under aerobic conditions (Warburg, 1926). This finding contradicted the Pasteur effect, named after Louis Pasteur, who found that in most mammalian cells the rate of glycolysis decreases significantly in the presence of oxygen. Glycolytic production of ATP under aerobic con- ditions, the Warburg effect, was found to be a characteristic of most cancer cells, and this finding was confirmed in various laboratories. Many cancer cell lines were also shown to rely on glycolysis for ATP production, and typically, the most glyco- lytic tumor cells were also found to be the most aggressive ones (Simonnet et al., 2002). Warburg originally assumed that aerobic glycolysis was a universal property of malignant cells due to defects in mitochondrial respiration, and he suggested that cancer might in fact be caused by impaired mitochondrial metabolism. In his lecture at the meeting of Nobel Laureates at Lindau, Lake Constance in June 1966, he stated: ‘‘Cancer, above all other diseases, has countless secondary causes. But, even for cancer, there is only one prime cause. Summarized in a few words, the prime cause of cancer is the replacement of the respiration of oxygen in normal body cells by a fermentation of sugar” (Warburg, 1967). He further hypothesized that moderate doses of ionizing radiation might reduce the activity of mitochondria in tumor cells below a threshold level essen- tial for cell survival, whereas mitochondria in normal cells would still be able to produce ATP, and that inhibition of oxidative phosphorylation could be a tool in cancer cell elimination. Indeed, in some forms of cancer, analysis of possible alterations in the oxidative phosphorylation machinery revealed down-regulation of the catalytic subunit of the mitochondrial ATP synthase (b-F1-ATPase) (Isidoro et al., 2005). Remarkably, the expression level of the b-F1-ATPase protein was found to inversely correlate with the rate of aerobic glycolysis. A link between mitochondrial dysfunction and upregulation of glycolysis could also be demonstrated in an in vitro model, when inhibition of oxidative phosphorylation by oligomycin in lung carcinoma cells was found to trigger a rapid increase in aerobic glycolysis. This observation suggests that cells might become glycolytic as a result of suppression of mitochondrial energy production (Lopez-Rios et al., 2007). Similarly, inhibition of respiration in two human lung cancer cell lines, H460 and A549, significantly upregulated glycolysis. In contrast, when glycolysis was suppressed, the cells were unable to sufficiently upregulate mitochondrial oxidative phosphorylation, which might have been a sign of mitochondrial dysfunction in these tumor cells (Wu et al., 2007). In contrast to these findings, other studies have demonstrated that tumor mitochondria are fairly functional with regards to respiration and ATP synthesis, exhibiting normal P/O and respiratory control ratios, and normal capabilities for the oxi- dation of respiratory substrates (Eakin et al., 1972). In fact, in some tumors, oxygen consumption was found to be similar, or even higher than in the normal tissue. Hence, in a critical analysis of Warburg’s theory, Sidney Weinhouse concluded: ‘‘no substantial evidence has been found that would indicate a respiratory defect, either in the machinery of electron trans- port, or in the coupling of respiration with ATP formation, or in the unique presence or absence of mitochondrial enzymes or cofactors involved in electron transport” (Weinhouse, 1976). In accordance with this, a comprehensive study of mitochon- drial function in slowly growing hepatomas showed almost no differences in respiratory parameters as compared to normal liver mitochondria (Pedersen et al., 1970). Mitochondria isolated from the ‘‘minimal deviation” hepatomas were also very similar in their electron transport activity. On the other hand, mitochondria isolated from rapidly growing hepatomas had diluted-appearing matrices and were incapable of oxidizing b-hydroxybutyrate, although oxidation of succinate oc- curred at rates comparable to, or even higher than in liver mitochondria. Mitochondria from these rapidly growing hepato- mas were able to phosphorylate added ADP, but they exhibited lower respiratory control ratio, indicative of increased proton permeability of the inner mitochondrial membrane (IMM). Thus, mitochondrial dysfunction can, indeed, be observed in tu- mors, particularly in the more aggressive and rapidly growing forms. When fast-growing tumors shift their ATP production towards glycolysis, mitochondrial activity slows down. Is this be- cause hypoxic conditions trigger destructive processes in the mitochondria, or do they remain functional after normalization of oxygen supply? In other words, is the Pasteur effect rather than the Warburg effect responsible for mitochondrial silencing (Zu and Guppy, 2004)? As mentioned above,
Details
-
File Typepdf
-
Upload Time-
-
Content LanguagesEnglish
-
Upload UserAnonymous/Not logged-in
-
File Pages15 Page
-
File Size-