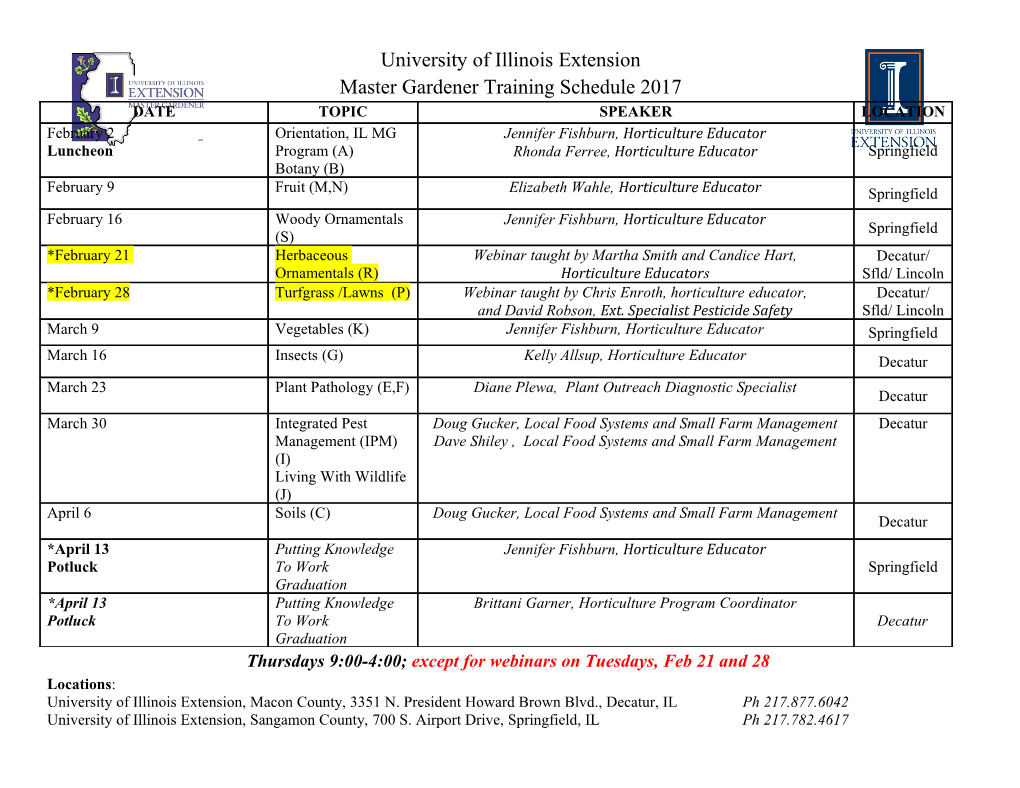
Optimization of a KATRIN source analysis tool and investigations of the potential to constrain the relic neutrino background Master Thesis of Florian Heizmann At the Department of Physics Institute of Experimental Nuclear Physics Reviewer: Prof. G. Drexlin Second reviewer: Prof. U. Husemann Advisor: Dr. K. Valerius Second advisor: Dr. M. Babutzka January 2015 KIT – University of the State of Baden-Wuerttemberg and National Research Center of the Helmholtz Association www.kit.edu ABSTRACT The KArlsruhe TRItium Neutrino (KATRIN) experiment { currently under con- struction at KIT { will determine the neutrino mass with an unprecedented sensi- tivity of 200 meV at 90 % C.L. by high-precision tritium β-decay spectroscopy. In order to reach this new level of neutrino mass sensitivity it is very important to understand the tritium source properties and the related systematic measurement uncertainties. Therefore, in the scope of this thesis, the electromagnetic design of a source analysis tool is optimized. Furthermore, the unique tritium source opens up the possibility to search for the elusive relic neutrinos, at least to set limits on the local relic neutrino overdensity, in a laboratory experiment. The potential of KATRIN to set those limits is also explored in this thesis. Das KArlsruhe TRItium Neutrino (KATRIN) Experiment { welches sich momen- tan im Aufbau am KIT befindet { ist konstruiert, um mittels hochaufl¨osender β- Zerfall Spektroskopie die Neutrinomasse mit einer bisher unerreichten Sensitivit¨at von 200 meV zu 90 % C.L. zu bestimmen. Das Erreichen dieses Sensitivit¨atsziels erfordert ein detailliertes Verst¨andnis der Eigenschaften der Quelle und der damit verbundenen systematischen Messunsicher- heiten. Im Rahmen dieser Arbeit wurde zu diesem Zweck das elektromagnetische Design eines Quellanalyseinstruments optimiert. Die einzigartige Tritiumquelle er- m¨oglicht zudem die Suche nach sogenannten Urknallneutrinos; zumindest eine obere Grenze fur¨ eine m¨ogliche lokale Uberdichte¨ des Neutrinohintergrundes sollte mit diesem Laborexperiment gesetzt werden k¨onnen. Das Potential von KATRIN, Mo- delle bezuglich¨ des Neutrinohintergrundes zu testen, wird in dieser Arbeit ebenfalls untersucht. iii CONTENTS Abstract iii 1. Introduction1 2. Neutrino physics3 2.1. The postulation & discovery of the neutrino..............3 2.2. Neutrinos in the Standard Model of particle physics..........5 2.3. Extension of the Standard Model of particle physics..........5 2.3.1. Neutrino oscillations.......................6 2.3.2. Neutrino mass generation.................... 10 2.4. Determination of the neutrino mass................... 13 2.4.1. Model-dependent measurements................. 13 2.4.2. Model-independent measurements................ 16 3. The KArlsruhe TRItium Neutrino Experiment 21 3.1. Neutrino Mass from tritium beta-decay................. 21 3.2. Components of the KATRIN experiment................ 22 3.2.1. Source section { WGTS..................... 22 3.2.2. Transport section { DPS and CPS............... 23 3.2.3. Spectrometer section { pre- and main spectrometer...... 24 3.2.4. Detector section.......................... 28 3.2.5. Rear Section............................ 28 3.3. Monitoring and impact of tritium source parameters.......... 28 4. Electromagnetic Design for the Rear Section of the KATRIN Experiment 31 4.1. Requirements for the electromagnetic design of the Rear Section... 31 4.2. Electromagnetic components....................... 32 4.2.1. Magnets.............................. 33 4.2.2. Electrodes............................. 36 4.3. Kassiopeia ................................ 40 4.3.1. Usage of Kassiopeia ...................... 40 4.3.2. Geometry definition........................ 40 4.3.3. Field computation........................ 41 4.3.4. Particle tracking......................... 44 v vi Contents 4.4. Simulations................................ 46 4.4.1. Tracking performance optimization............... 46 4.4.2. Performance tests......................... 49 4.4.3. Optimization of electron gun parameters............ 50 4.5. Summary................................. 53 5. Cosmic Neutrino Background 57 5.1. Theory of the cosmic neutrino background............... 58 5.1.1. Cosmic microwave background cosmology........... 58 5.1.2. Cosmic neutrino background cosmology............. 61 5.1.3. Detection of cosmic neutrino background............ 65 5.2. Signal identification of the cosmic neutrino background at KATRIN. 69 5.2.1. Energy resolution......................... 70 5.2.2. Doppler effect........................... 71 5.2.3. Final state distribution...................... 71 5.3. Signal implementation of the cosmic neutrino background....... 73 5.3.1. SSC and KaFit ......................... 74 5.3.2. Statistical methods........................ 75 5.3.3. FSD implementation....................... 77 5.3.4. Implementation challenges.................... 78 5.3.5. Implementation result...................... 79 5.4. Sensitivity of KATRIN for measuring the cosmic neutrino background 80 5.4.1. Sensitivity for the cosmic neutrino background overdensity.. 81 5.4.2. Sensitivity for the neutrino mass................ 82 5.4.3. Cosmic neutrino background overdensity systematics..... 83 5.4.4. Discussion of the results..................... 84 5.5. Summary................................. 86 6. Conclusions & Outlook 89 A. Rear Section geometry definitions of components 91 A.1. Beam tube geometry definition..................... 91 A.2. E-gun geometry definition........................ 93 A.3. Post acceleration geometry definitions.................. 97 A.4. Electric dipole electrodes geometry definitions............. 99 A.5. Solenoid geometry definitions...................... 100 A.6. Magnetic dipoles geometry definitions.................. 102 B. Rear Section assembly of components 105 B.1. Rear Section complete geometry..................... 105 B.2. Rear Section object properties...................... 107 C. Rear Section simulation 113 D. Rear Section settings 121 Bibliography 131 List of Acronyms 139 vi Contents vii Acknowledgements 141 vii CHAPTER 1 INTRODUCTION Since the postulation of the neutrino in 1930 [PKW64] and its first detection in 1953 [RC53], neutrino physics has developed into an active and continuously growing research field. Since then, many properties of these particles have been measured. The present neutrino model consists of three active neutrinos, participating only in the weak interaction due to the fact that they carry neither electric nor color charge. Oscillation experiments showed that there is evidence for a yet unknown but nonzero neutrino mass [F+98,A +01]. The strongest neutrino mass limits today come from tritium β-decay experiments at Mainz and Troitsk [Oli14]. The KArlsruhe TRItium Neutrino (KATRIN) experiment aims to measure the neu- trino mass with unprecedented sensitivity of 200 meV at 90 % C.L. The main foun- dations for this factor 10 improvement in neutrino mass sensitivity with respect to previous experiments are an ultra-luminous tritium source combined with high pre- cision β spectroscopy. Since the observable in β-decay experiments is the squared neutrino mass, this requires an overall improvement of statistical and systematic uncertainties of a factor 100. In order to achieve this goal, the properties of the KATRIN source and transport section (STS) will be continuously measured by the calibration and monitoring systems (CMS). As part of the CMS, the so-called \Rear Section" is designated to serve as a versatile tritium source analysis tool. To enable various calibration procedures for the KATRIN experiment the Rear Sec- tion must provide an electron beam with precisely known characteristics. The prop- erties of this beam strongly depend on the electromagnetic design (EMD) of the Rear Section. Therefore, previous EMD simulations are re-evaluated and extended in this thesis by the implementation of the Rear Section into the most recent KATRIN particle tracking software Kassiopeia. Furthermore, the large amount of tritium in the gaseous source enables the search for relic neutrinos forming a cosmic neutrino background (CνB), the neutrino pendant to the cosmic microwave background (CMB). This CνB originates 1 s after the Big Bang, providing a time window very close to the origin of the universe. Today, these neutrinos are non-relativistic due to their rest mass, motivating models of relic neutrino clustering [RW04] which may result in a local relic neutrino overdensity. Up to now, the most promising method for relic neutrino detection seems to be 1 2 1. Introduction induced β-decay [KFM10], since the process does not involve a kinematic threshold. Therefore, the KATRIN experiment with its ultra-luminous tritium source { though not a dedicated CνB experiment { will be in a prime position to constrain the local relic neutrino overdensity, as will be shown in this thesis. In chapter2, a brief introduction to neutrino physics is given. It recapitulates the status of this broad field of particle physics, including the role of the neutrinos in the Standard Model of particle physics, neutrino oscillations and recent neutrino mass experiments. Chapter3 gives an overview of the next generation neutrino mass experiment KATRIN. Briefly, the measuring principle of KATRIN and its main components are explained. Furthermore, the purpose and role of the source analysis tool Rear Section in the context of the KATRIN experiment is outlined. After introducing the Rear Section, its EMD properties are discussed in chapter4.
Details
-
File Typepdf
-
Upload Time-
-
Content LanguagesEnglish
-
Upload UserAnonymous/Not logged-in
-
File Pages151 Page
-
File Size-