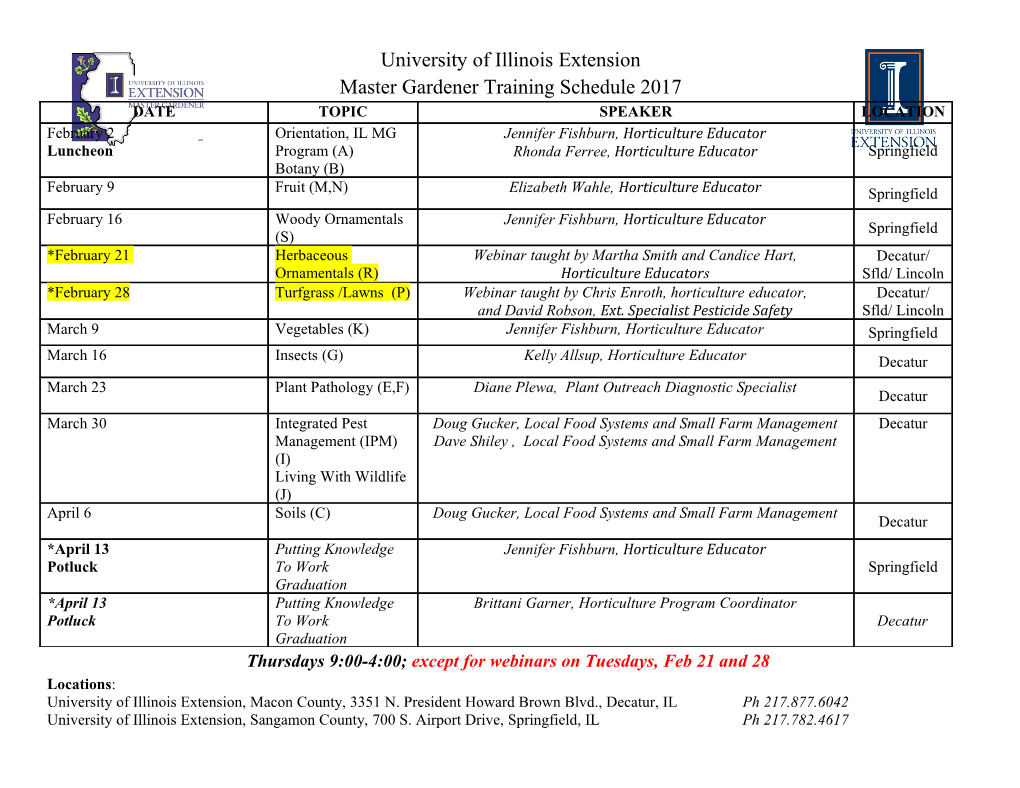
Dynamic Modeling of Multi Stage Flash (MSF) Desalination Plant by Hala Faisal Al-Fulaij Department of Chemical Engineering University College London Supervisors: Professor David Bogle Thesis submitted for the degree of Doctor of Philosophy (Ph.D.) at University College London (UCL) July 2011 I, Hala Faisal Al-Fulaij, confirm that the work presented in this thesis is my own. Where information has been derived from other sources, I confirm that this has been indicated in the thesis. Hala F. Al-Fulaij Acknowledgments i Acknowledgments This Ph.D. was carried out between March 2007 and January 2011 at the Chemical Engineering department, University College London (UCL). This work was supervised by Professor David Bogle (UCL) and Professor Hisham Ettouney (Kuwait University). I take this opportunity to thank both of my supervisors for general guidance throughout the project and their great help, support and insight. I also thank Professor Giorgio Micale and Doctor Andrea Cipollina from University of Palermo who expended their time and made significant contribution to my knowledge especially in the computer tools field. Also, I am grateful for the love and support of my family, especially my mother, father, husband and sisters. Their patience and encouragement have given me the strength to complete my thesis study. Finally I would like to dedicate this thesis to my lovely children (Rayan, Maryam, AbdulWahab and Najat) hoping them the most of health, success, and happiness. Abstract ii Abstract The world population is increasing at a very rapid rate while the natural water resources remain constant. During the past decades industrial desalination (reverse osmosis (RO) and multistage flash desalination (MSF)) became a viable, economical, and sustainable source of fresh water throughout the world. In the MSF units, the flashing of seawater involves formation of pure vapour, which flows through a wire mesh demister to remove the entrained brine droplets and then condenses into product water. The study presented in this thesis is motivated by the absence of detailed modelling and analysis of the dynamics of the MSF process and the demister. A detailed dynamic model can be used in design, control, startup/shutdown and troubleshooting. Most of the previous studies on MSF plant focused on model development and presented limited amount of performance data without any validation against plant data. Literature models of the MSF demister are either empirical or semi-empirical. This motivated use of a computational fluid dynamics (CFD) software to design a new demister that will reduce the pressure/temperature drop in the vapour stream without affecting the separation efficiency of brine droplets and allows the optimal design of complete MSF units. Lumped parameter dynamic models were developed for the once through (MSF-OT) and the brine circulation (MSF-BC) processes. The models were coded using the gPROMS modelling program. The model predictions for both MSF-OT and MSF-BC in steady state and dynamic conditions showed good agreement against data from existing MSF plants with an error less than 1.5%. Dynamic analysis was made to study plant performance upon making step variations in system manipulated variables and identify stable operating regimes. New stable operating regimes were reached upon changing the cooling water flow rate by + 15% and increasing the recycle brine flow rate by 15% and decreasing it by 7%. This was not the case for the steam temperature where its variation was limited to + 2-3 %. This behavior is consistent with the actual plant data. The FLUENT software was used to model the MSF demister using different combinations of Eulerian and Lagrangian approaches to model the vapour and the Abstract iii brine droplets. This provided the open literature with novel and new methodologies for design and simulation of the MSF demister using CFD. A new demister design was made upon varying the wire diameter. This led to an efficient design with low pressure drop and high separation efficiency. This design was used in the MSF/gPROMS model to predict its effect on the heat transfer area. The new design provided reductions of 3-39% in the condenser heat transfer area without affecting dynamic performance. Since the tubing system accounts for almost 70% of the capital cost, then this would reduce the plant capital cost and product unit cost. The modelling approach presented in this thesis enables design of thermal desalination units to determine optimal heat transfer area and optimized operating conditions. Table of Contents iv Table of Contents Acknowledgments i Abstract ii List of Figures ix List of Tables xvii Chapter 1: Introduction and motivation 1 Chapter 2: Water Desalination 5 2.1 Introduction 5 2.2 Water Shortage Problem 5 2.3 Sources of Fresh Water 7 2.4 Types of water 9 2.5 Sea Water Composition and Properties 10 2.6 Need for Water Desalination 12 2.7 Classification of Desalination Technology 18 2.7.1 Thermal Processes 18 2.7.2 Membrane Processes 19 2.8 Multistage Flash Desalination 19 2.8.1 Once through MSF Process 21 2.8.2 Brine circulation MSF Process 23 2.8.3 Comparison between MSF Processes 28 2.8.4 Flashing stage description 30 2.9 Conclusion 34 Chapter 3: Modeling of MSF Processes: Literature Review 35 3.1 Introduction 35 3.2 Simple Mathematical Models 36 3.3 Detailed Mathematical Models 40 Table of Contents v 3.3.1 Steady State Models 40 3.3.2 Dynamic Models 44 3.4 Conclusion 51 Chapter 4: Dynamic Modeling of MSF Plant 53 4.1 Introduction 53 4.2 Model Basis and Assumptions 54 4.3 Model Structure 55 4.4 Once Through MSF Process (MSF-OT) 56 4.4.1 Mathematical equations 56 4.4.1.1 Lower Level Model (Flashing Stage Model) 57 4.4.1.2 Higher Level Model (MSF Plant) 65 4.5 Brine circulation MSF Process (MSF-BC) 69 4.5.1 Mathematical equations 69 4.5.1.1 Lower Level Model (Heat Gain Section Falshing Stage Model) 69 4.5.1.2 Lower Level Model (Heat Rejection Section Falshing Stage Model) 71 4.5.1.3 Higher Level Model 73 4.6 gPROMS Modeling Language 73 4.7 Conclusion 75 Chapter 5: Validation and Results of Dynamic Modeling of MSF Plants 77 5.1 Introduction 77 5.2 Modeling real MSF-OT Plants 77 5.2.1 Cases investigated, assignment and initial conditions 77 5.2.2 Model Validation Results 78 5.2.2.1 Steady state Validation Results 79 5.2.2.2 Dynamic Model Validation Results 81 5.2.3 Dynamic Response Results 85 5.3 Modeling Real MSF-BC Plants 90 Table of Contents vi 5.3.1 Cases investigated, assignment and initial conditions 90 5.3.2 Model Validation Results 92 5.3.2.1 Steady state Validation Results 93 5.3.2.2 Dynamic Model Validation Results 94 5.3.3 Dynamic Response Results 97 5.3.4 Effect of demister losses on heat transfer area of the condenser tubes 113 5.4 Conclusion 116 Chapter 6: CFD Modeling of the Demister 117 6.1 Introduction 117 6.2 Demisters Element Description 121 6.3 Modeling of Demisters : Literature Review 129 6.4 Mathematical Model Equations (Eulerian-Eulerian method) 132 6.4.1 Porous Media with Multi Phase Flow 133 6.4.2 Tube Banks with Multi Phase Flow 143 6.5 Mathematical Model Equations (Eulerian-Lagrangian method) 147 6.5.1 Tube Banks with Discrete Phase Model 147 6.6 Description of the CFD Code 151 6.7 Model Assumptions 153 6.7.1 Porous Media model 154 6.7.2 Tube Banks- Multi phase model 154 6.7.3 Tube Banks with discrete phase model 154 6.8 Solution Methods of CFD Codes 155 6.9 Conclusion 156 Chapter 7: Validation and Results of CFD Modeling of the Demister 158 7.1 Introduction 158 7.2 Porous Media – Multi Phase Model Approach: 158 Table of Contents vii 7.2.1 Grid sensitivity analysis 159 7.2.2 Cases investigated, geometries and boundary conditions 167 7.2.3 Model validation 169 7.2.4 Modeling results and discussion 175 7.3 Tube Banks –Multi Phase Model Approach 177 7.3.1 Grid sensitivity analysis 178 7.3.2 Cases investigated, geometries and boundary conditions 184 7.3.3 Model validation 185 7.4 Tube Banks –Discrete Phase Model Approach 191 7.4.1 Grid sensitivity analysis 191 7.4.2 Cases investigated, geometries and boundary conditions 196 7.4.3 Model validation 199 7.4.4 Comparison between performance of different demisters 207 7.5 Conclusion 209 Chapter 8: Improving MSF Plant Performance 211 8.1 Introduction 211 8.2 Effect of Improved Demister on the MSF-OT Plant 212 8.2.1 Effect of new demister on the product quality 212 8.2.2 Effect of new demister on the flashing stage condenser area 213 8.3 Effect of Improved Demister on the MSF-BC 217 8.3.1 Effect of new demister on the product quality 217 8.3.2 Effect of new demister on the flashing stage condenser area 218 8.3.3 Effect of new demister system dynamics 226 8.4 Conclusion 229 Table of Contents viii Chapter 9: Conclusions and Future work 230 9.1 Conclusions 230 9.2 Future work 233 Notation 237 Bibliography 242 Publications 254 Appendix A: Model Physical Properties Correlations 256 Appendix B: Degree of Freedon in gPROMS Models 263 Appendix C: (MSF-OT) gPROMS Code 267 Appendix D: (MSF-BC) gPROMS Code 286 List of Figures ix List of Figures Figure 2.1 Variation in world population from 1823 to 2050 6 Figure 2.2 Desalination market shares of large producers 13 Figure 2.3 MSF unit capacity growth 14 Figure 2.4 Market share of the main desalination process for desalination of river, brackish and seawater 15 Figure 2.5 Market share of the main desalination process for desalination of seawater 15 Figure 2.6 Cumulative production capacity of MSF plants in the Gulf countries 16 Figure 2.7 Conventional thermal and membrane desalination processes 18 Figure 2.8 View of a typical multistage flash desalination plant 23 Figure 2.9 Multistage flash desalination once through process (MSF- OT) 24 Figure 2.10 Multistage flash desalination with brine circulation (MSF- BC) 27 Figure 2.11 MSF flashing stage showing input and output variables 33 Figure 2.12 Two types of MSF orifices.
Details
-
File Typepdf
-
Upload Time-
-
Content LanguagesEnglish
-
Upload UserAnonymous/Not logged-in
-
File Pages334 Page
-
File Size-