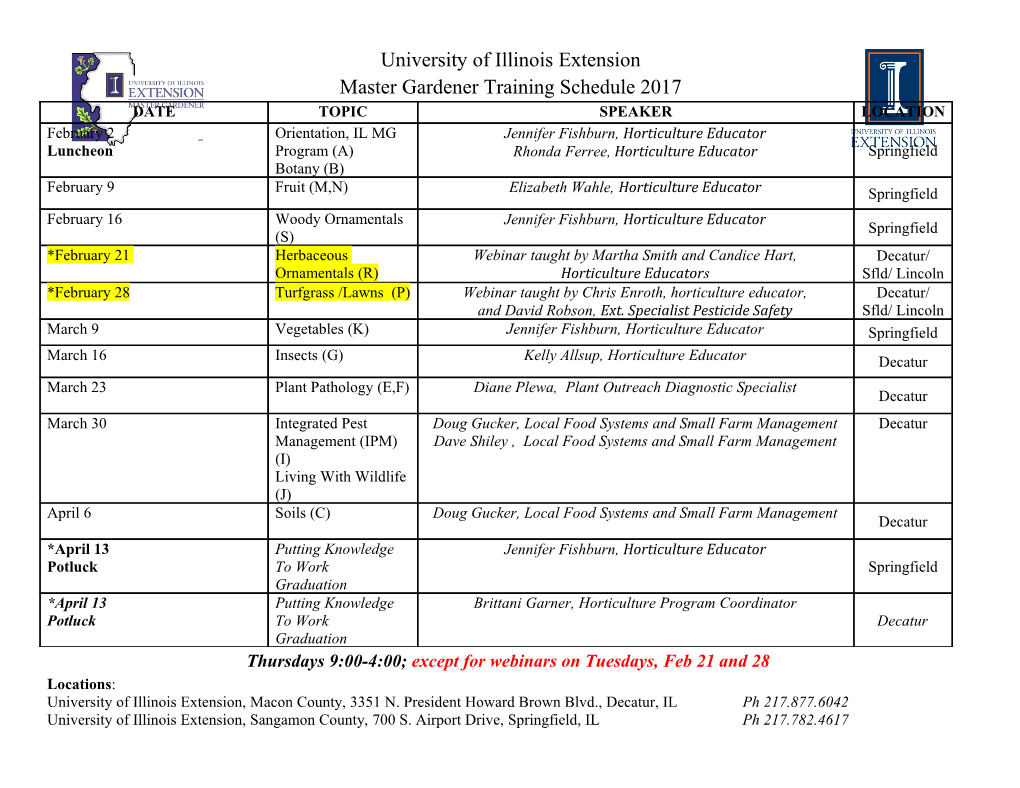
1 In memory of Mario Govi and Ugo Zezza DEDICATED To Fulvio, Vera, Paolo and Francesca. 2 TABLE OF CONTENTS Signature page ……………………………………………………………………………………..1 Dedication ……………………………………………………………………………………...…..2 Table of contents ………………………………………………………………………………..…3 List of Figures ………………………………………………………………………………….….5 List of Tables ……………………………………………………………………….….………..…7 Acknowledgements ……………………………………………………..………………..………..8 I. Introduction ……………………………………………………………………………….…….9 Reference cited ………………………………………………………………………...…12 II. Sesia Fossil section, magmatic plumbing of a large Permian caldera exposed to a depth of 25 km Geologic setting …………………………………………………………………….….....15 Reconstruction of the pre-alpine crustal section ……………………………………....…17 Geochronology …………………………………………………………………………...20 Discussion ……………………………………………………………………….………..37 Conclusions ……………………………………………………………………………...39 Reference cited …………………………………………………………………………...39 III. Structure and eruptive history of Sesia Caldera ………………………………..……………46 Geologic framework ………………………………………………………….…….…….46 Methods …………………………………………………………………………….…….52 Geology of Sesia caldera ……………………………………………………………..….54 Overview: Sesia Caldera …………………………………………………………………70 Comparison with other calderas ……………………………………………………….…80 Reference cited …………………………………………………………………………...80 IV. Hydrothermal circulation in Sesia caldera …………………………………………...………96 Introduction ………………………………………………………………………...….…96 Geological framework ………………………………………………………….…..…….97 Methods …………………………………………………………………………..………98 3 Results ……………………………………………………………………………………99 Discussion …………………………………………………………………………….…104 Conclusions ………………………………………………………………………..……109 Acknowledgements ..………………...…………………………………………..………110 Reference cited ………………………………………………………………………….133 V. Conclusions and perspectives ……………………………….………………………..…...…135 Riassunto ………………………………………………………………………….…….138 Reference cited ………………………………………………………………………….139 4 LIST OF FIGURES Figure 2.1 ……………………………………...…………………………...………….….……..16 Figure 2.2 ……………………………………...……………………………………….…..…….19 Figure 2.3 ……………………………………...…………………………………….….….…….21 Figure 2.4 ……………………………………...…………………………………….…...….…..23 Figure 2.5 ……………………………………...…………………………………….…...….…..25 Figure 2.6 ……………………………………...…………………………………………….…...26 Figure 2.7 ……………………………………...……………………………………….…….….28 Figure 2.8 ……………………………………...………………………………….………….….29 Figure 2.9 ……………………………………...…………………………………….……….….30 Figure 2.10 ……………………………………...………………….………………..……….….32 Figure 2.11 ……………………………………...……………………………….…..……….….33 Figure 2.12 ……………………………………...………………………………..….……….….36 Figure 2.13 ……………………………………...……………………………….…..……….….38 Figure 3.1 ……………………………………...…………………………………………….…..48 Figure 3.2 ……………………………………...…………………………………………….…..58 Figure 3.3 ……………………………………...………………………………………………...66 Figure 3.4 ……………………………………...…………………………………………….…..67 Figure 3.5 ……………………………………...…………………………………………….…..69 Figure 3.6 ……………………………………...…………………………………………….…..73 Figure 3.7 ……………………………………...…………………………………………….…..78 Figure 3.8 ……………………………………...…………………………………………….…..88 Figure 3.9 ……………………………………...…………………………………………….…..89 Figure 3.10 ……………………………………...………………….……………….……….…..90 Figure 3.11 ……………………………………...……………………………….….……….…..91 Figure 4.1 ……………………………………...………………………………………….…….111 Figure 4.2 ……………………………………...………………………………………….…….112 Figure 4.3 ……………………………………...…………………………………………..……113 Figure 4.4 ……………………………………...…………………………………………………114 Figure 4.5 ……………………………………...…………………………...…………………….116 Figure 4.6 ……………………………………...……………………………...………………….117 Figure 4.7 ……………………………………...………………………………...……………….118 Figure 4.8 ……………………………………...…………………………………...…………….119 5 Figure 4.9 ……………………………………...……………………………………...………….120 Figure 4.10 ……………………………………...………………….……………….…..………..121 Figure 4.11 ……………………………………...……………………………….….……..……..122 Figure 4.12 ……………………………………...……………………………….….………..…..123 6 LIST OF TABLES Table 2.1 ……………………………………...……………………………………………….33 Table 2.2 ……………………………………...……………………………………………….36 Table 2.3 ……………………………………...……………………………………………….38 Table 3.1 ……………………………………...……………………………………………….92 Table 3.2 ……………………………………...……………………………………………….94 Table 3.3 ……………………………………...……………………………………………….95 Table 4.1 ……………………………………...……………………………………………….124 Table 4.2 ……………………………………...……………………………………………….126 Table 4.3 ……………………………………...……………………………………………….127 Table 4.4 ……………………………………...………………………………………...……..128 Table 4.5 ……………………………………...………………………………………...……..131 7 AKNOWLEGMENTS I would like to thank my PhD advisor Silvano Sinigoi to give me the opportunity to work together on rocks of the Sesia fossil section. I would like to thank James E. Quick, that discovered the caldera, to have suggested the research topic, to have helped to settle the problem, to have visited several times my field area, and to have been a source of critical advices and support in this research. I would like to thank Peter W. Lipman to have accepted as a field assistant for three summers, to have mentored me into the study of fossil calderas and to have visited my field area. From the discussion with him showed up lots of the ideas of this thesis. His example and advices changed my vision of rocks. I would like to thank William C. McIntosh, to encourage me, for useful discussion, for important age data on those rock and to visit my field area. I would like to thank I. Richards ad R.T. Gregory for the work on oxygen isotopes and for the useful discussion. I would like to thank Angelo De Min for all the help and the useful discussion about the genesis of those rocks. I would like to thank Joe L. Wooden, Gabriella Peressini, Gabriella Demarchi for the great opportunity to collaborate with them. These interactions have resulted in a research paper published in Geology, included in this dissertation. The text in Chapter II, in full, is taken from Geology, v. 37, no. 7, p. 603-606; Quick, J. E., Sinigoi, S., Peressini, G., Demarchi, G., Wooden, J. L., and Sbisa, A., 2009, Magmatic plumbing of a large Permian caldera exposed to a depth of 25 km. Thanks for the financial support provided by MIUR Project PRIN 2007 to S. Sinigoi. Thanks to Lisa A. Morgan to have introduced me to the geology of Yellowstone-Snake River Plain. Thanks the phd “collegio” of the Università di Trieste, and specially the “responsabile”, Peter Suhadolc. Thanks to Davide Lenaz to have helped me in reviewing this manuscript while I wasn’t able to read it any more. Thanks to Franco Cucchi e Alessio Mereu for the support and thoughtful advices on GIS. Thanks to Filippo Parisi to have shared with me the office for three years, his presence was a cool breeze. Special thank to all friends from Valsesia and specially to the Franco Cameroni and his family, Aldo Tocchio and Mirko Zucchetto. I would like to thank my parents Vera e Paolo, my brother Fulvio and my partner Francesca. 8 Chapter I: Introduction Caldera-forming eruptions rank among the most violent geologic events, Earth’s largest calderas form as the ground collapses during immense volcanic eruptions, when hundreds to thousands of cubic kilometers of magma are explosively withdrawn from the Earth’s crust over a period of days to weeks. Understanding the mechanics of those magmatic systems is critical issue for our society, since it is extremely desiderable to forecast largest class volcanic eruption (Lowenstern et al., 2006), and being able to predict and prevent the disastrous effects at the continental scale and their consequences on climatic changes (Rampino, 2002; Self, 2006; Sparks et al., 2005). Our understanding of these events is incomplete and its infancy mainly due to the lack of directly observed eruptions. Consequently it is challenging to recognize the difference of signals between smaller and larger eruptions. Notably models for smaller explosive eruptions, that had been directly observed and studied, are sometimes inadequate to explain in detail eruption dynamics (Castro and Dingwell, 2009). The development of an appropriate model is the foundation in order to recognize meaning versus meaningless signals of incoming eruption and to forecast diverse modes and magnitude. Today models of the magmatic systems are based on inferences from the petrology of the eruption products and are constrained by geophysical observations (Bachmann et al., 2007). We are still lacking in constrains on the basic assumptions of those models, such as observations of on a single system in which all its elements are exposed. To our knowledge the preferred model is that the eruptive material of a large explosive eruption is the crystal mush, that stores previously the eruption beneath 5 km depth, while in case of crystal poor units the melt is extracted from the same crystal mush that acts as a sponge (Bachmann and Bergantz, 2004; Bachmann et al., 2002). Despite those models, we know that large volume with >10% melt underneath a caldera have never been imaged geophysically at 5 km depth (DeNosaquo et al., 2009; Husen et al., 2004) (Guidarelli et al., 2006; Weiland et al., 1995) and ignimbrite erupt with a high percentage of melt, ranging from >90% to 55% of melt. In this dissertation I argue that what we need to test he basic constrains of those models, because if part of the eruptible material is stored deeper, or alternatively the only melt upraises during eruption from a deeper reservoir, the signals relative to 5-7 km may be not significant until the eruption started. Despite the fact that both geophysical studies on active calderas and petrochemical studies of eruptive products have investigated the problem in detail, our understanding
Details
-
File Typepdf
-
Upload Time-
-
Content LanguagesEnglish
-
Upload UserAnonymous/Not logged-in
-
File Pages141 Page
-
File Size-