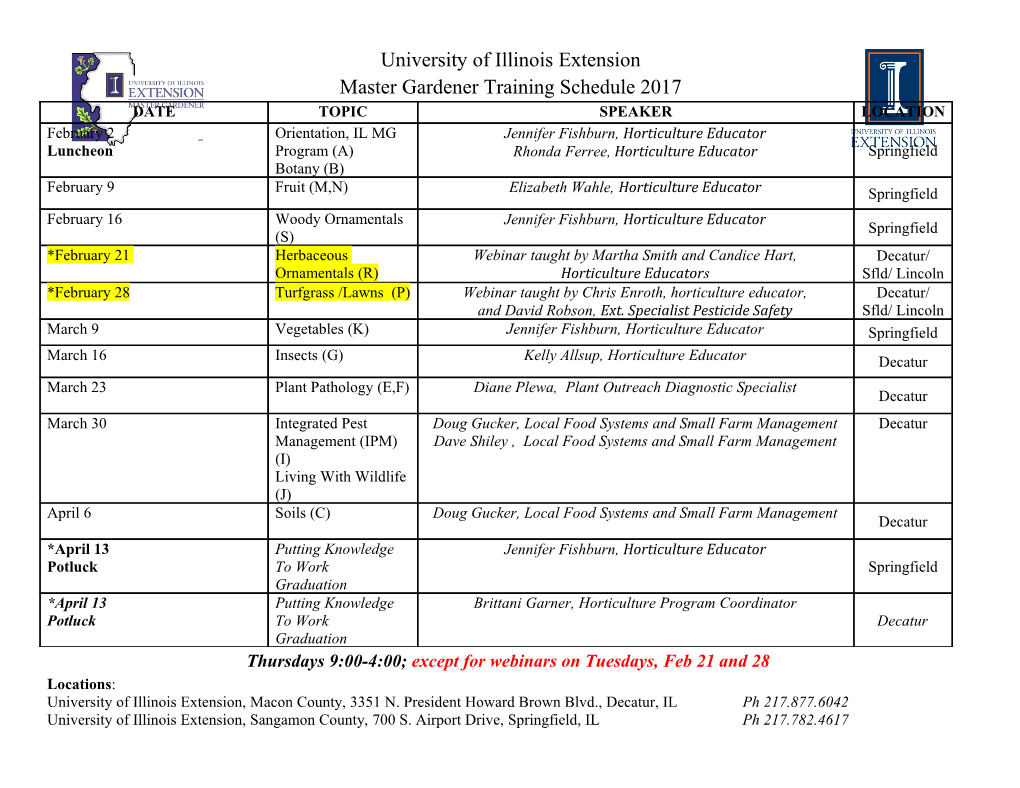
Bull Volcanol (2001) 63:227–237 DOI 10.1007/s004450100137 RESEARCH ARTICLE Richard J. Stevenson † · Donald B. Dingwell Nikolai S. Bagdassarov · Curtis R. Manley Measurement and implication of “effective” viscosity for rhyolite flow emplacement Received: 6 August 2000 / Accepted: 10 February 2001 / Published online: 17 May 2001 © Springer-Verlag 2001 Abstract Hazardous explosive activity may sporadically ing model to construct thermal and viscosity profiles re- accompany the extrusion of silicic lava domes. Model- vealing conditions during the emplacement of the Ben ling of the emplacement of silicic domes is therefore an Lomond lava dome. important task for volcanic hazard assessment. Such modelling has been hampered by a lack of a sufficiently Keywords Rhyolite · Lava flow · Viscosity · Pumice · accurate rheological database for silicic lavas with crys- Arrhenius dependence · Cooling model · Vesiculation tals and vesicles. In the present study, the parallel-plate viscometry method was applied to determine the shear viscosity of five natural rhyolitic samples from a vertical Introduction section through the Ben Lomond lava dome, Taupo Volcanic Centre, New Zealand. Rheological measure- The emplacement of silicic lava flows and of the Pacific ments were performed at volcanologically relevant tem- Rim over the past decade has focused interest and atten- peratures (780–950°C) and strain rates (10–5–10–7 s–1). tion on the potential hazards associated with these volca- Although these samples are in the metastable state, vis- noes. Explosive activity may precede or accompany the cosity determinations, melt composition, as well as water extrusion of lava. The distribution of vesicular textures and crystal contents of samples were demonstrably sta- on silicic lava flows has been related to their likelihood ble during experiments. For samples containing up to of experiencing explosive behaviour during flow ad- 5 vol.% microlites, the composition of the melt, rather vance (Fink and Manley 1989; Fink et al. 1992), and than the physical effect of suspended crystals, had great- variations in vesicularity are, in part, related to variations er influence on the effective viscosity of the silicic mag- in volatile contents (Westrich et al. 1988; Anderson ma. Samples with 10 vol.% microlites and containing a and Fink 1990). Some lava flows contain explosion cra- flow banding defined by microlites show no significant ters indicating high internal pressures in these flows orientational effects on apparent viscosity. The rheologi- (Anderson and Fink 1992; Fink et al. 1992), caused by cal measurements were used together with a simple cool- crystallisation and the resultant build-up of excess pres- sure in the interior of the flow (Sparks 1997). Also, Editorial responsibility: T.H. Druitt flow-front collapse can generate pyroclastic flows † Deceased (Anderson and Fink 1990; Anderson et al. 1995; Jousset R.J. Stevenson · D.B. Dingwell and Okada 1999; Matthews et al. 1997; Mothes et al. Bayerisches Geoinstitut, Universität Bayreuth, 1998; Richter et al. 1994; Swanson and Holcomb 1990; 95440 Bayreuth Germany Wadge et al. 1995; Watanabe et al. 1999; Yamashina and N.S. Bagdassarov (✉) Shimizu 1999), as observed at active dacitic lava domes Institut für Meteorologie und Geophysik, such as Lascar and Socompa volcanoes in northern J.W. Goethe Universität-Frankfurt, Feldbergstrasse 47, Chile, Usu–Hokkaido and Unzen in Japan, Cotopaxi in 60323, Frankfurt am Main, Germany e-mail: [email protected] Ecuador, Santiaguito in Guatemala, Mount Drum in Tel.: +49-69-79823376, Fax: +49-69-7982380 Alaska, and Mount St. Helens in the U.S. The formation of explosion pits and pyroclastic flows are the two most C.R. Manley 52 158th Place NE, Bellevue, WA 98008–4314 USA significant volcanic hazards associated with viscous si- licic lava flows. Present address: D.B. Dingwell, Institut für Mineralogie, Despite its obvious importance for risk assessment, Petrologie und Geochemie, Universität München, previous modelling of silicic flows has been hampered Theresienstrasse 47/III, 80333 Munich, Germany by an insufficient rheological database on lavas for input 228 into eruption models. Also, very recently, questions have been raised concerning the accuracy of empirical models which predict the effect of water on the viscosity of rhyolitic and andesitic melts (Dingwell et al. 1996; Richet et al. 1996; Hess and Dingwell 1996; Schulz et al. 1996; Scaillet et al. 1996). As a first approximation, some physical properties can be deduced from detailed physical and textural studies of lava flow carapaces (Fink 1983) or from sections bisecting the thickness of flows (Stevenson et al. 1994). More recently, however, viscosity has been measured for natural rhyolitic obsidi- ans at volcanologically relevant temperatures and strain rates (Stevenson et al. 1995, 1996). This new type of da- ta permits much more accurate modelling of the rheolog- ical properties of silicic flows. We studied a silicic lava dome in New Zealand, the Ben Lomond dome, Taupo Volcanic Centre. Viscosity measurements on natural samples taken from specific la- va flow facies, together with a simple cooling model, were used to constrain the timing of formation of the textural units. These results are useful for volcanic haz- ards assessment for this and other, similar calc-alkaline lava flows. The Ben Lomond flow The Ben Lomond dome (ca. 100 ka) consists of two flow lobes up to 3.5 km in length, bisected by a fault scarp that exposes up to two-thirds of the internal flow stratigraphy (Fig. 1a). This lava flow consists of an eroded finely ve- sicular pumice carapace (Fig. 1b, FVP), an upper obsidi- an layer (U.OBS) cross-cut by a pumicious breccia and a spherulitic transition zone (TZ) into crystalline rhyolite (RHY; for more details see Stevenson et al. 1994). The base of the flow is not exposed, as valleys between flow lobes are filled by pumice alluvium. Almost all the FVP layer has been eroded, and the flow is covered by a 22-ka ignimbrite. Near the margins of the flow lobes, flow lay- ering ramps upward, steepening in dip from 30 to 80° to- ward the surface. The inferred vent position is near the summit of the lava dome, based on flow banding orienta- tion and the surface slope of the lava lobes. This flow was chosen for rheological modelling be- cause it: (a) has been adequately documented in the liter- ature in terms of its stratigraphy (Stevenson et al. 1994) and its composition and microstructural features (Sharp et al. 1996); (b) contains both near-surface and internal sections of the flow; and, most importantly, (c) it con- tains fresh non-hydrated, unweathered rhyolitic obsidian ranging from aphyric to containing ca. 10 vol.% microl- Fig. 1 Morphology of A the Ben Lomond Dome and B a strati- ites, making it well suited to high-precision viscosity graphic column with sampling sites. FVP finely vesicular pumice; U. OBS upper obsidian layer; TZ spherulitic transition zone; measurements using the parallel-plate method. RHY crystalline rhyolite layer. Stratigraphic units are in metres (redrawn from Stevenson et al. 1994) Sample description Viscosity measurements were performed on five natural samples: one pumice (BLP) and four obsidians (BL3, 4, 229 6, 7). The stratigraphic locations of these samples are Preparation of annealed and hydrated samples shown in Fig. 1b. In addition, viscosity measurements were performed on one sample (BL6-r) re-fused at In order to prepare an essentially dry rhyolite melt, chips 1650°C to remove water, and one sample hydrothermally (ca. 5 mm size) of BL6 were vesiculated at 1300°C for a treated so as to contain 0.5 wt.% H2O. These experi- few minutes followed by cooling and crushing to a fine ments were necessary in order to better constrain the vis- grain powder. The powder was incrementally annealed in cosity of the melt phase with varying water contents for a Pt-crucible for several hours at 1650°C followed by the vesicular pumiceous samples. slow cooling to room temperature over 24 h (Stevenson et al. 1998). The end product (BL6-r) contained no crys- tals, no bubbles, and had a water content of ca. Natural samples 0.03 wt.% measured by FTIR. This formed the starting material for the synthesis of a hydrous BL6 melt. Pow- Sample BL7 is a platy obsidian clast collected from FVP dered BL6-r was added to a Pt-capsule, the equivalent of (finely vesicular pumice) within the exposed upper 6 m 0.5 wt.% distilled water was added, and then the capsule of the lava flow carapace. Individual clasts are less than was sealed by arc-welding. The capsule was examined 0.36 m size and comprise aphyric, non-spherulitic black for possible leakage by testing for weight loss after dry- obsidian with striated surfaces and rare bubble-rich inter- ing in an oven at 150°C for at least 1 h. Oven drying also nal layers. Microlite content is <0.2 vol.% consisting of ensured that the water (as vapour) was evenly distributed strings of Fe–Ti oxides and opx–cpx rods. throughout the capsule. The hydrated melt was synthe- A black aphyric obsidian (sample BL6) was collected sised in an internally heated pressure vessel (CRSCM, within the upper 5 m of the upper obsidian layer Orléans, France) at 1300°C in excess of 3 kbar pressure (U. OBS in Fig. 1b). This sample is sparsely spherulitic for ca. 65 h (for method details see Roux et al. 1994). and microlite-poor with <0.5 vol.% microlites of rod-like The end product was a bubble-free glass. All samples shape (10×1 µm size), stringer and spider-like form. Rare were held at 100°C during at least 1 h before rheological microphenocrysts (<40 µm width) occur comprising experiments in order to remove the absorbed water. magnetite, augite, amphibole and tabular plagioclase laths of 60×30 µm average size.
Details
-
File Typepdf
-
Upload Time-
-
Content LanguagesEnglish
-
Upload UserAnonymous/Not logged-in
-
File Pages11 Page
-
File Size-