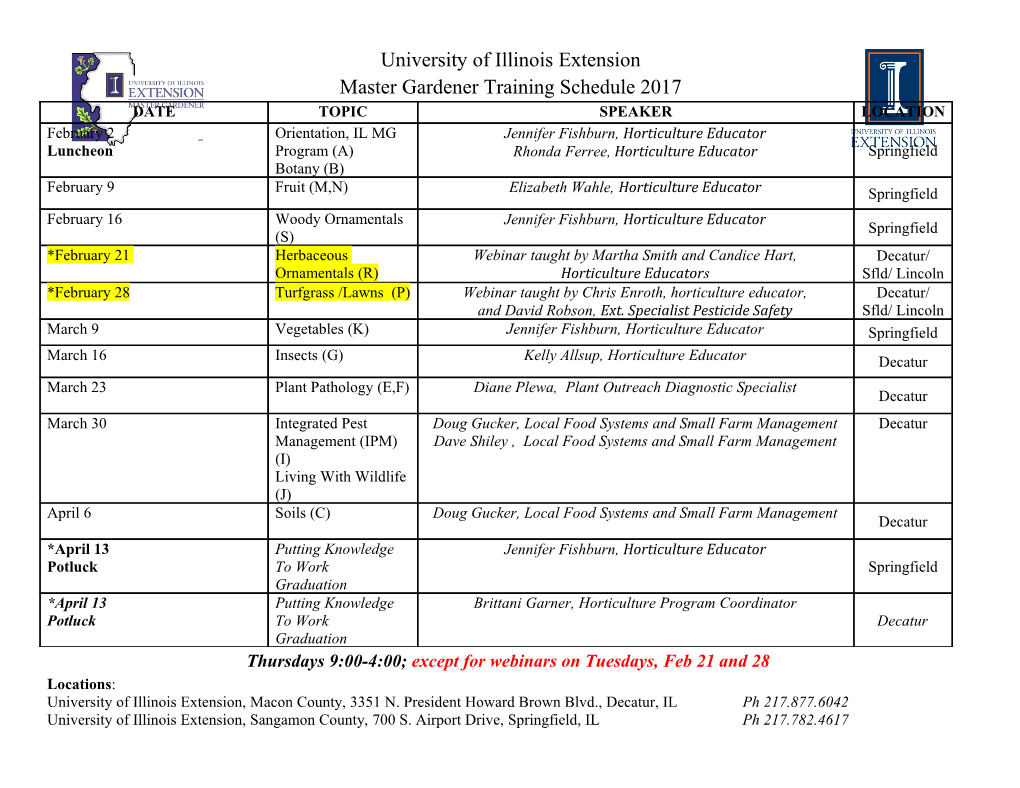
Bacteria Harnessing Complexity By Eshel Ben Jacob, Yakir Aharonov and Yoash Shapira School of Physics and Astronomy Raymond & Beverly Sackler Faculty of Exact Sciences The Maguy-Glass Chair in Physics of Complex Systems Tel Aviv University, 69978 Tel Aviv Israel Abstract The study of bacterial colonies - groups of bacteria grown from a single or few similar cells - is a crucial step towards understanding biofilms, which are composed of many different bacterial colonies. In this article, we review some of the exciting discoveries about the cooperative behavior of bacteria in colonies, which might shed new light on biocomplexity in general and biofilms in particular. The review is aimed at researchers from different disciplines - microbiology, biology, chemistry, physics, mathematics, and computer science. To make the presentation comprehensible to such a wide audience, we avoided the use of specialized terminology of the different disciplines and limited the experimental and computational details. We start with the realization that, under natural growth conditions, bacteria can self- 9 12 organize into hierarchically structured colonies, 10 -10 bacteria each. To that end, they developed and utilize a great variety of biochemical communication agents, such as simple molecules, polymers, peptides, complex proteins, genetic material, and also "cassettes of genetic information" like plasmids and viruses. Complex colonial forms (patterns) emerge through the communication-based singular interplay between individual bacteria (the micro-level) and the colony (the macro-level). Each bacterium is, by itself, a biotic autonomous system with its own internal cellular gel that possesses informatics capabilities (storage, processing and interpretation of information). These afford the cell certain freedom to select its response to biochemical messages it receives, including self-alteration and broadcasting messages to initiate alterations in other bacteria. Such self-plasticity and decision-making capabilities elevate the level of bacterial cooperation during colonial self-organization. As the individuals increase their adaptability to the group, the colony elevates its durability and adaptability to the environment by increasing its complexity. The essential new lesson learned from bacteria is that colonial higher complexity provides the degree of plasticity and flexibility required for better durability and adaptability of the whole colony to a dynamic environment. According to this picture, new features collectively emerge during biotic self-organization on every level, from the internal cellular gel to the whole colony. The 1 cells thus assume newly co-generated traits and abilities that are not explicitly stored in the genetic information of the individuals. For example, bacteria cannot genetically store all the information required for creating the colonial patterns. In the new picture, they don't need to, since the required information is cooperatively generated as self-organization proceeds by bacterial communication, informatics and self-plasticity capabilities. Thus, the bacteria need only have genetically stored the guidelines for producing these capabilities and using them to generate new information as required. We illustrate these special capabilities by exposing the bacteria to non-lethal levels of antibiotics. We also demonstrate bacterial ability to sense the effect of weak electromagnetic (EM) signals on aqueous solutions. The observations illustrate that bacteria use their intracellular flexibility, involving signal transduction networks and genomic plasticity, to collectively maintain self and shared interpretations of chemical cues, exchange of meaning-bearing chemical messages, and dialogues. The meaning-based communication permits the formation of colonial intentional behavior, purposeful alteration of colony structure and decision-making - features we might begin to associate with bacterial social intelligence. Such social intelligence, should it exist, would require going beyond communication to encompass additional intracellular processes, yet unknown, for generating inheritable colonial memory and commonly shared genomic context. Introduction Bacteria, being the first form of life on earth, had to devise ways to synthesize the complex organic molecules required for life. They are able to reverse the spontaneous course of entropy increase and convert high-entropy inorganic substances into low- entropy life-sustaining molecules. Three and a half billion years have passed, and the existence of higher organisms depends on this unique bacterial know-how. Even for us, with all our scientific knowledge and technological advances, the ways bacteria solve this fundamental requirement for life is still a mystery [1-7] We do know that this is not a solitary endeavor for the bacteria, and under natural conditions they employ chemical communication to form hierarchically structured colonies, 109-1012 bacteria each [4-33]. By acting jointly, they can make use of any available source of energy and imbalances in any environments, from deep inside the earth crust to nuclear reactors and from freezing icebergs to sulfuric hot springs; and they can convert any available substances, from tar to metals. 2 Under unpredictable hostile environmental conditions, when the odds are against survival, the bacteria turn to a wide range of strategies for adaptable collective responses. These cooperative modes of behavior are manifested through remarkable different patterns formed during colonial self-organization. The aesthetic beauty of these geometrical patterns [34,35] is striking evidence of an ongoing cooperation that enables the bacteria to achieve a proper balance of individuality and sociality as they battle for survival, while utilizing pattern-formation mechanisms that we have only recently begun to understand. The geometrical patterns can also be associated with quantified measures, as explained in Appendix A. Yet, the naked eye can distinguish between patterns better than the most advanced image processing methods. Therefore, in this article we will rely on the reader’s geometrical perception in comparing between the geometrical patterns generated by the bacterial colonies. In bacterial self-organization, unlike inanimate pattern formation [36-39], there is an additional inherent degree of plasticity: the building blocks of the colony are themselves living organisms, each with internal degrees of freedom, internally stored information and internal assessment of external chemical messages. These afford each bacterium freedom to respond flexibly and even alter itself, by means of modifying its genetic expression patterns. At the same time, efficient adaptation of the colony to adverse growth conditions requires self-organization on all levels – which can only be achieved via cooperative behavior of the individual cells. For that purpose, bacteria communicate by a broad repertoire of biochemical agents. At the same time, each bacterium has equally intricate intracellular communication mechanisms involving, for example, signal transduction networks [40,41]. These are used to generate intrinsic meaning for contextual interpretations of the chemical messages and for formulating appropriate responses [13]. Biochemical messages are also used in bacterial linguistic communication for exchange of meaningful information across colonies of different species, and even with other organisms [42]. As we are discovering, bacterial communication-based cooperation encompasses colony morphogenesis, which includes coordinated gene expression, regulated cell 3 differentiation and division of tasks. Collectively, bacteria can glean information from the environment and from other organisms, interpret the information (assign meaning), develop common knowledge, and learn from past experience. The colony behaves much like a multicellular organism, or even a social community with elevated complexity and plasticity that afford better adaptability to whatever growth conditions might be encountered [5]. Branching Patterns of Lubricating Bacteria To illustrate the ability of bacteria to cope with conflicting environmental constraints, we begin with the branching patterns exhibited by the Paenibacillus dendritiformis lubricating bacteria [11]. This class of bacteria developed a special strategy to move on hard surfaces – they collectively excrete special chemicals to form a layer of lubricant in which they can swim. As they swim, they push the layer forward, paving their own way. A dilemma arises when, in addition, the available food is not sufficient to sustain a dense population [43-48]. When such conditions are mimicked in Petri-dishes with hard substrates of low nutrient concentration, new and interesting phenomena are observed, as shown in Fig 1. Collectively, the bacteria turn on genes to emit chemicals (surfactants) used to extract fluid from the substrate, thus creating on top of the crust a lubricating layer with a well defined envelope within which they can swim. The task is not simple, as the production of the lubricant requires collective action of dense bacterial population which the food- depleted substrate can not sustain. The solution comes in the form of a branching structure of the colony – within each branch the bacterial density is sufficiently high, yet the average population density of the colony is sufficiently low to match the availability of food. The real trick is the careful adjustment of the lubricant viscosity and its production
Details
-
File Typepdf
-
Upload Time-
-
Content LanguagesEnglish
-
Upload UserAnonymous/Not logged-in
-
File Pages49 Page
-
File Size-