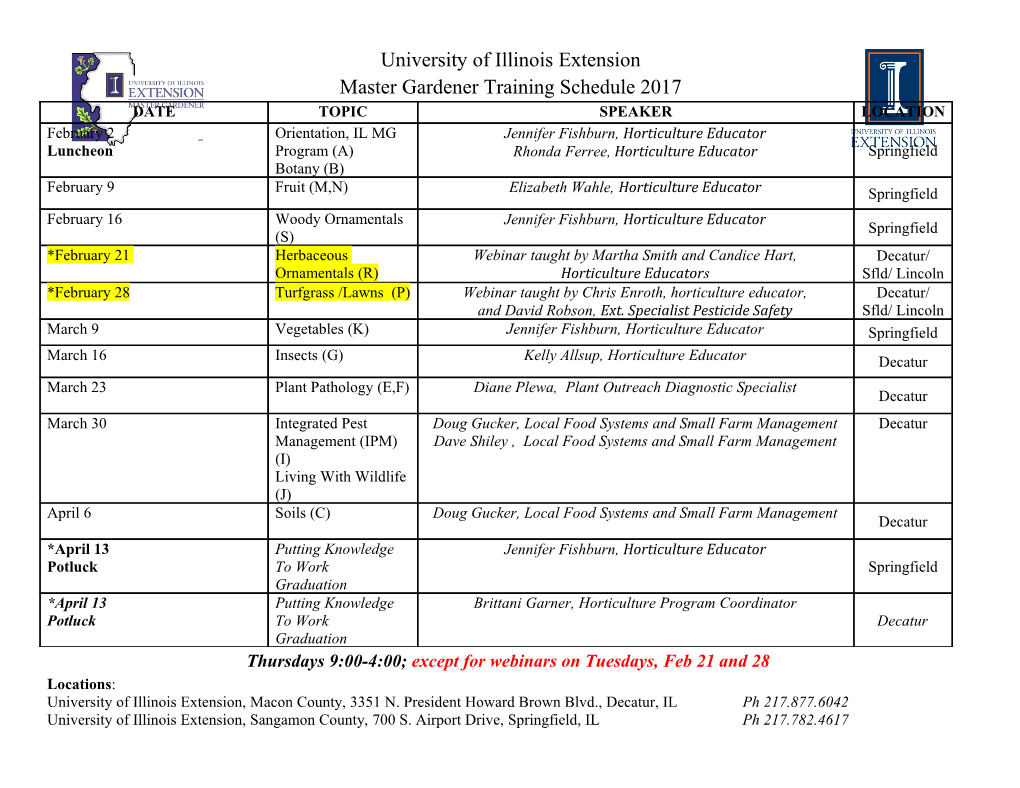
Assembly of large-area, highly ordered, crack-free inverse opal films Benjamin Hattona,b, Lidiya Mishchenkoa, Stan Davisc, Kenneth H. Sandhagec,d, and Joanna Aizenberga,b,1 aSchool of Engineering and Applied Sciences, Harvard University, Cambridge, MA 02138; bWyss Institute for Biologically Inspired Engineering, Harvard University, Cambridge, MA 02138; cSchool of Materials Science and Engineering, Georgia Institute of Technology, Atlanta, GA 30332; and dSchool of Chemistry and Biochemistry, Georgia Institute of Technology, Atlanta, GA 30332 Edited by Jack Halpern, The University of Chicago, Chicago, IL, and approved April 16, 2010 (received for review January 24, 2010) Whereas considerable interest exists in self-assembly of well- templates have been produced via sedimentation (1), shear flow ordered, porous “inverse opal” structures for optical, electronic, (4), spin-coating (17), and evaporative (18–20) or “flow- and (bio)chemical applications, uncontrolled defect formation controlled” deposition (21, 22). The use of these self-assembly has limited the scale-up and practicality of such approaches. Here processes to form large-area colloidal crystal films has very we demonstrate a new method for assembling highly ordered, typically resulted in the formation of cracks, domain boundaries, crack-free inverse opal films over a centimeter scale. Multilayered colloid vacancies, and other defects. Efforts have also been composite colloidal crystal films have been generated via evapora- made to inhibit cracking (23) and control domain orientation tive deposition of polymeric colloidal spheres suspended within a of colloidal crystal films through changes of evaporative deposi- hydrolyzed silicate sol-gel precursor solution. The coassembly of a tion conditions (20, 24), particle presintering (25), and deposition sacrificial colloidal template with a matrix material avoids the need onto topologically patterned substrates (26). In the second step, for liquid infiltration into the preassembled colloidal crystal and sol-gel solutions have been used as matrix precursors for the minimizes the associated cracking and inhomogeneities of the re- syntheses of porous oxide materials, such as SiO2, TiO2, and sulting inverse opal films. We discuss the underlying mechanisms Al2O3 (1–5). Matrices have also been deposited via infiltration that may account for the formation of large-area defect-free films, of polymer precursors (6, 27), salt precursors (28), nanoparticles their unique preferential growth along the h110i direction and (29), or vapor phase precursors (7, 30, 31). Capillary forces unusual fracture behavior. We demonstrate that this coassembly generated during drying after self-assembly, or infiltration of approach allows the fabrication of hierarchical structures not the template, have led to additional cracking of these mechani- achievable by conventional methods, such as multilayered films cally fragile templates. Efforts to increase the strength of self- and deposition onto patterned or curved surfaces. These robust assembled templates have been made by partial sintering (32) SiO2 inverse opals can be transformed into various materials that or growth of necks between spheres (33). Moreover, excessive retain the morphology and order of the original films, as exempli- infiltration and deposition in the second fabrication step results fied by the reactive conversion into Si or TiO2 replicas. We show in overlayer formation, whereas incomplete or nonconformal that colloidal coassembly is available for a range of organometallic deposition often leads to structural collapse during template sol-gel and polymer matrix precursors, and represents a simple, removal in the third step. Whereas layer-by-layer vapor phase low-cost, scalable method for generating high-quality, chemically processes, such as atomic layer deposition, may yield continuous tailorable inverse opal films for a variety of applications. and conformal coatings and avoid the formation of cracks asso- ciated with liquid infiltration or drying, these approaches are coassembly ∣ colloidal assembly ∣ crack-free films ∣ inverse opals ∣ relatively time consuming and expensive, and still require a de- nanoporous fect-free colloidal template to grow upon. No reliable, low-cost, scalable methods have been demonstrated for the synthesis of elf-assembly methods have attracted considerable interest as crack-free, robust inverse opal films comprised of single-crystal Spotential alternatives to conventional top-down processes for domains over large (cm or more) lateral dimensions. the scalable, low-cost syntheses of nanoporous solid structures. Herein we demonstrate the evaporative coassembly of a sacri- Examples include the self-assembly of periodic colloidal “opal” ficial colloidal template with a matrix material in a single step to templates that, in turn, can direct the infiltration and deposition yield a colloidal composite, thereby avoiding the need for liquid B of functional materials to yield nanoporous “inverse opal” struc- infiltration into a preassembled porous structure (Fig. 1 ). tures (1–5). Inverse opals can exhibit a high degree of intercon- Previously, colloidal coassembly of structures has been achieved – nected porosity (approximately 75%) with extremely uniform size with binary mixtures of colloids (34 38), supramolecular mesos- (average size normally in the range of 100–1000 nm) and periodic tructures (39), and with a combination of supramolecular and distributions of pores, achieved through colloidal monodispersity. colloidal self-assembly (40). Also, sol-gel silicates have been “ ” Such structures have been shown to be potentially useful in a wide added as a temporary glue for SiO2 colloids (41). Our approach range of fields, including photonics (6–10), tissue engineering goes beyond the coassembly of binary colloidal mixtures or a (11, 12), sensing (13, 14), and catalysis (10, 15, 16). However, combination of supramolecular and colloidal self-assembly, whereas conventional self-assembly has yielded ordered inverse and relies on polymer colloids [e.g., polystyrene (PS) or poly opal structures over modest (≤10 μm) length scales, such pro- (methyl methacrylate) (PMMA)] assembling in a broad range cesses have been plagued by uncontrolled formation of defects over larger length scales (2, 3), thus limiting their real-world Author contributions: B.H., K.H.S., and J.A. designed research; B.H., L.M., S.D., and J.A. applications. performed research; L.M., B.H., K.H.S., and J.A. analyzed data; and B.H., L.M., K.H.S., Conventional colloidal self-assembly of inverse opal materials and J.A. wrote the paper. (Fig. 1A) has generally been conducted in three sequential steps: The authors declare no conflict of interest. 1. assembly of a colloidal crystal template (e.g., comprised of This article is a PNAS Direct Submission. polymer latex spheres), 2. infiltration and deposition of a matrix Freely available online through the PNAS open access option. phase, or a precursor to a solid matrix phase, and 3. selective 1To whom correspondence should be addressed. E-mail: [email protected]. removal of the colloidal crystal template to yield an inverse This article contains supporting information online at www.pnas.org/lookup/suppl/ porous structure (1–5). In the first step, thin film colloidal crystal doi:10.1073/pnas.1000954107/-/DCSupplemental. 10354–10359 ∣ PNAS ∣ June 8, 2010 ∣ vol. 107 ∣ no. 23 www.pnas.org/cgi/doi/10.1073/pnas.1000954107 Fig. 2. SEM images of colloidal and inverse opal films. (A) PMMA colloidal crystal film (as synthesized), showing cracks propagating along the close- SCIENCES 111 – μ “ ” packed f g planes and spaced at the 5 10 m scale); (B) I-SiO2 (inverse opal) Fig. 1. Schematics of conventional colloidal template self-assembly (A), APPLIED PHYSICAL film by sol-gel infiltration (calcined to remove PMMA template), showing an and coassembly of colloids with a soluble matrix precursor (B), for the synth- overlayer coating, cracking in both the inverse opal and overlayer coating, eses of inverse opal thin films. The conventional method typically involves the sequential steps of colloidal self-assembly, matrix infiltration, and template and a region of SiO2 infiltration into a preexisting template defect (white removal. The use of such conventional colloidal self-assembly to generate arrow); (C) I-SiO2 film by PMMA/sol-gel coassembly, showing highly uniform films with no cracks. Note that the thickness of the coassembled film is large-area films has been plagued with the formation of defects, such as comparable (and higher) than the thickness of the cracked film shown in overlayer coatings, multiple domains, and significant cracking. Colloidal coas- (A). Left scale bars 10 μm, right scale bars 1 μm. sembly combines the steps of template self-assembly with matrix infiltration ¼ ¼ into one process in which colloids are allowed to assemble directly from the sol-gel solution, to yield robust inverse opal films with no overlayer, very free films were deposited. Further additions of TEOS to the large (mm to cm) ordered domains, and no cracks, due to the “gluing” action deposition mixture (>0.20 mL TEOS solution per 20 mL PMMA of the sol-gel matrix. suspension) caused the initial formation of an overlayer, which increased in thickness with further TEOS additions. of sol-gel precursor solutions (e.g., MðÞ OH4 ,M¼ Si, Ti, Ge) Fig. 2 compares the quality of the coassembled I-SiO2 films through an evaporative deposition (18, 19, 42), to form a with the PMMA colloidal crystals
Details
-
File Typepdf
-
Upload Time-
-
Content LanguagesEnglish
-
Upload UserAnonymous/Not logged-in
-
File Pages6 Page
-
File Size-