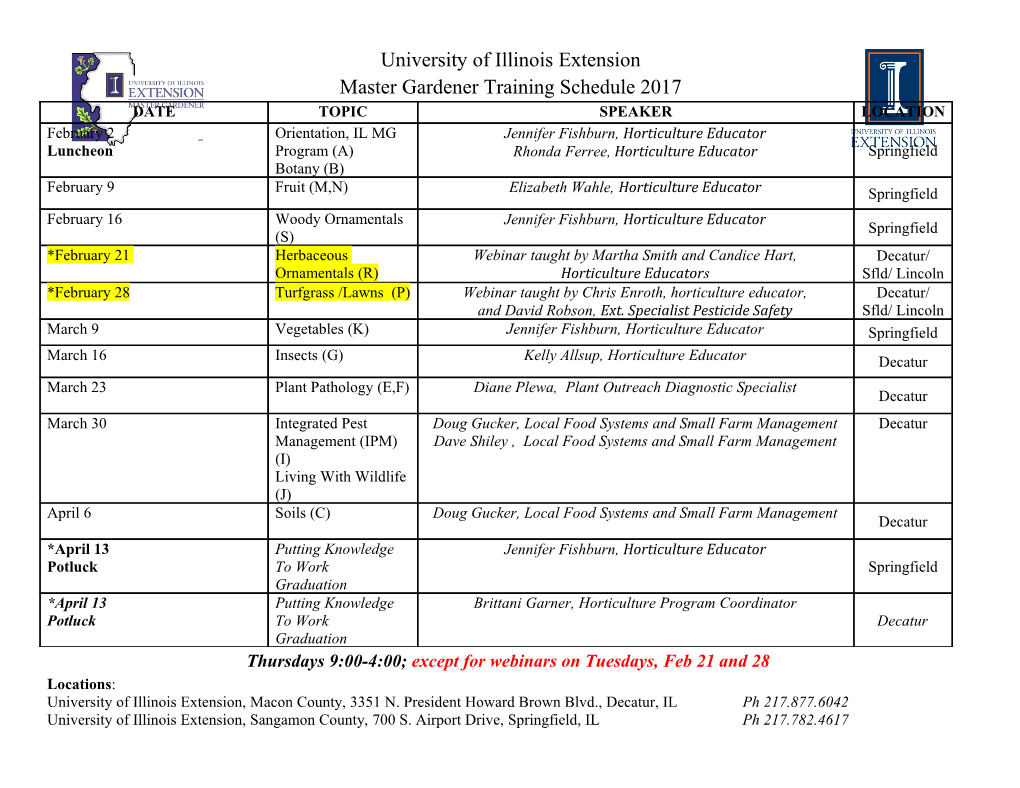
Stellar evolution The structure of a star gravity 300,000 earth masses How do stars work? • Gravity wants to collapse star ⇒ Star heats up ⇒ Pressure increases ⇒ Pressure force stops the collapse How do stars work? • Gravity wants to collapse star ⇒ Star heats up ⇒ Pressure increases ⇒ Pressure force stops the collapse gravity pressure • “Hydrostatic equilibrium”: ⇒ Hottest and densest in center ⇒ Highest pressure in center How do stars work? • Gravity wants to collapse star ⇒ Star heats up ⇒ Pressure increases ⇒ Pressure force stops the collapse lower pressure • “Hydrostatic equilibrium”: ⇒ Hottest and densest in center net force ⇒ Highest pressure in center higher pressure “Buoyancy” How do stars work? • Gravity wants to collapse star ⇒ Star heats up ⇒ Pressure increases ⇒ Pressure force stops the collapse • “Hydrostatic equilibrium”: ⇒ Hottest and densest in center ⇒ Highest pressure in center “Buoyancy” How do stars work? • Gravity wants to collapse star ⇒ Star heats up ⇒ Pressure increases ⇒ Pressure force stops the collapse • “Hydrostatic equilibrium”: ⇒ Hottest and densest in center ⇒ Highest pressure in center Pressure stratification The structure of a star gravity pressure 30 million degrees F 12,000 degrees F Artist’s rendition But stars radiate! • Anything with temperature > 0 radiates ⇒ Stars lose heat and cool gravity Their pressure should decrease They should contract under gravity As they contract, they heat back up pressure ⇒ With just gravity: stars “slowly” shrink lifetime for the sun (“Kelvin-Helmholtz”): solar age t ~ 15 million years But stars radiate! • Anything with temperature > 0 radiates ⇒ Stars lose heat and cool Their pressure should decrease They should contract under gravity As they contract, they heat back up ⇒ With just gravity: stars “slowly” shrink lifetime for the sun (“Kelvin-Helmholtz”): solar age t ~ 15 million years Actual solar age: • t > 1 billion years (fossils) Solution: Heating • Sun, like rest of universe, is made mostly of hydrogen • Four hydrogen nuclei can fuse into one helium nucleus ( + stuff ) • Einstein (1905): E = mc2 Solution: Heating • Sun, like rest of universe, is made mostly of hydrogen • Four hydrogen nuclei can fuse into one helium nucleus ( + stuff ) • Einstein (1905): E = mc2 4 x mH > mHE ΔE = 0.8% mc2 • 4xH→He fusion releases energy! (Eddington 1926) • Fusion reactions heat stars (Bethe, Chandrasekhar 1939) Solution: Heating • Stellar equilibrium: gravity Gravity balanced by pressure Radiation balanced by fusion pressure Artist’s rendition Main sequence stars Most stars we see are burning • high mass hydrogen • This makes them all similar: Stellar structure mostly determined by stellar mass Mass determines temperature and luminosity low mass “Main sequence” The stellar “main sequence”: They evolve slowly Determined by mass (not along the main sequence) Main sequence stars • What happens when fuel runs out? For sun: tfuel ~ 10 billion years More massive stars burn more quickly Once hydrogen is gone, collapse? Main sequence stars • We could burn 3 x Helium to 1 x Carbon... • We could burn 1 x Carbon + 1 x Helium to 1 x Oxygen... • Can we go on like this forever? It becomes harder to fuse (requires more pressure) Gain energy until we hit iron Above iron: net energynet loss net energy gain it takes net energy to fuse Iron is the end of the line! So: Without fuel... • Stars lose heat and cool Their pressure should decrease They should contract under gravity As they contract, they heat back up Then cool some more... ⇒ With just gravity: stars collapse ⇒ Where does it stop? Does any other form of pressure appear? Does the star collapse to R<RS Quantum degeneracy • Particles are social animals: Pauli exclusion principle • “Bosons” like each other Photons Easy to cram them into tight spaces • “Fermions” don’t like each other Electrons, neutrons Each particle requires some space Quantum “degeneracy” pressure, independent of temperature! White dwarfs: After sufficient compression: Degenerate matter • (helium, carbon, oxygen) Normal gas (50 km thick) Electrons become “degenerate”, (too close together) 5000 km Resist further compression No more contraction Size comparison with regular stars No more burning (stops A carbon white dwarf: at carbon or oxygen) The largest diamond possible Just cooling New equilibrium where gravity is balanced by degeneracy pressure a white dwarf Neutron stars More mass = more gravity proton electron • + - • More gravity = smaller star • Denser neutron electrons get crammed into protons no electrons = no degeneracy pressure collapse! • All matter converted to neutrons • Neutron degeneracy pressure neutron star • Neutrons take less space 10 km radius A neutron star: A giant atomic nucleus Neutron stars • White dwarf more massive than 1.4 suns: Collapse Supernova explosion Formation of neutron star • Neutron are little magnets Neutron stars are super-magnets! ~ 1 trillion times stronger than typical bar magnet Neutron stars • Exact structure of neutron stars unknown For 1.4 Msun: R ~ 10 km Compare: Rs ~ 4.5 km Neutron stars • Exact structure of neutron stars unknown black hole limit Complicate particle physics But we know: More massive = smaller Upper mass limit: ~ 3 solar masses Then: Black hole! • How to make a black hole: Take a very massive star and let it run out of fuel How massive? About 40 solar masses. How long does this take? About 10 million years. The fate of a dying star gravity <12 white dwarf (a carbon or oxygen ball) The fate of a dying star >12 neutron star (a neutron ball) The fate of a dying star >40 black hole (an “event horizon”) Stellar mass black holes • Now we know that massive stars make black holes • Black hole mass: A few solar masses • But how do we find such black holes? Gravity on other star? Light bending? Radiation? Most stars are “binaries” • They have a stellar companion • They orbit each other following Kepler’s laws • Most massive stars should have a companion • Massive stars evolve into black holes Some hold on to their companions when they make black holes ⇒ Some black holes have stellar companions Accretion • When a black hole comes close enough: It can syphon off matter from the companion star! This matter must ultimately fall into the black hole This process is called accretion • This is how black holes grow • This is how we find and study most black holes.
Details
-
File Typepdf
-
Upload Time-
-
Content LanguagesEnglish
-
Upload UserAnonymous/Not logged-in
-
File Pages33 Page
-
File Size-