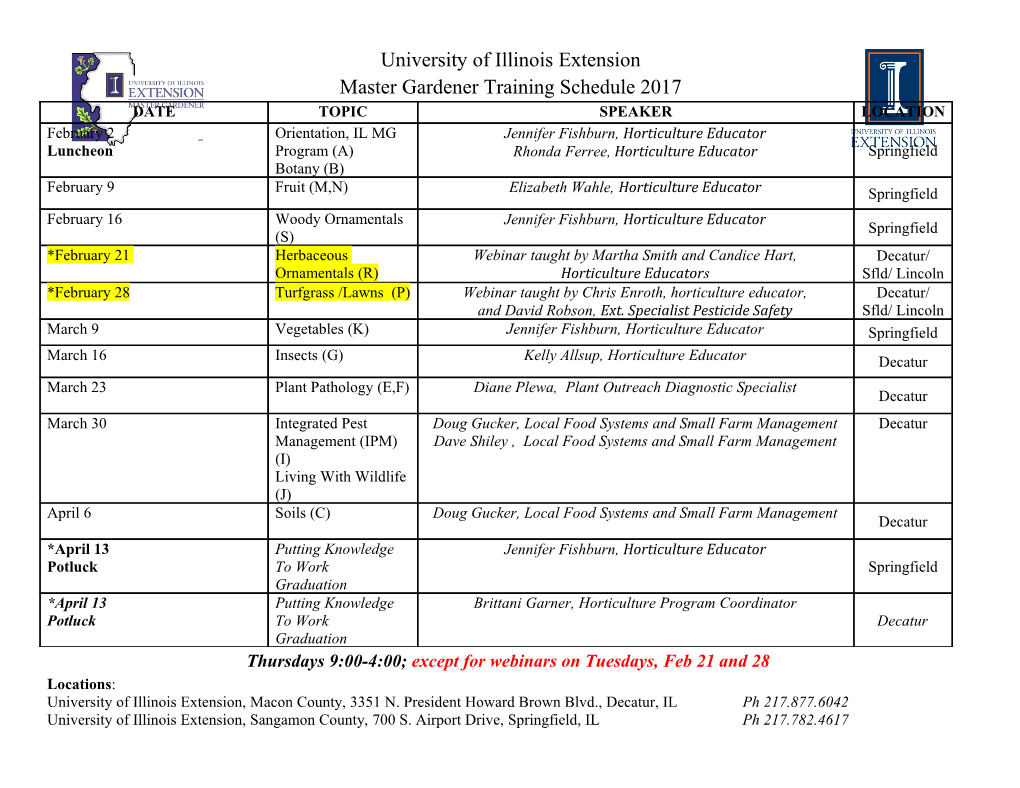
Chapter 04 Rotational Motion P. J. Grandinetti Chem. 4300 P. J. Grandinetti Chapter 04: Rotational Motion Angular Momentum Angular momentum of particle with respect to origin, O, is given by l⃗ = ⃗r × p⃗ Rate of change of angular momentum is given z by cross product of ⃗r with applied force. p m dl⃗ dp⃗ = ⃗r × = ⃗r × F⃗ = ⃗휏 r dt dt O y Cross product is defined as applied torque, ⃗휏. x Unlike linear momentum, angular momentum depends on origin choice. P. J. Grandinetti Chapter 04: Rotational Motion Conservation of Angular Momentum Consider system of N Particles z m5 m 2 Rate of change of angular momentum is m3 ⃗ ∑N l⃗ ∑N ⃗ m1 dL d 훼 dp훼 = = ⃗r훼 × dt dt dt 훼=1 훼=1 y which becomes m4 x ⃗ ∑N dL ⃗ net = ⃗r훼 × F dt 훼 Total angular momentum is 훼=1 ∑N ∑N ⃗ ⃗ L = l훼 = ⃗r훼 × p⃗훼 훼=1 훼=1 P. J. Grandinetti Chapter 04: Rotational Motion Conservation of Angular Momentum ⃗ ∑N dL ⃗ net = ⃗r훼 × F dt 훼 훼=1 Taking an earlier expression for a system of particles from chapter 1 ∑N ⃗ net ⃗ ext ⃗ F훼 = F훼 + f훼훽 훽=1 훽≠훼 we obtain ⃗ ∑N ∑N ∑N dL ⃗ ext ⃗ = ⃗r훼 × F + ⃗r훼 × f훼훽 dt 훼 훼=1 훼=1 훽=1 훽≠훼 and then obtain 0 > ⃗ ∑N ∑N ∑N dL ⃗ ext ⃗ rd ⃗ ⃗ = ⃗r훼 × F + ⃗r훼 × f훼훽 double sum disappears from Newton’s 3 law (f = *f ) dt 훼 12 21 훼=1 훼=1 훽=1 훽≠훼 P. J. Grandinetti Chapter 04: Rotational Motion Conservation of Angular Momentum ⃗ ∑N ∑N dL ⃗ ext = ⃗r훼 × F = ⃗휏훼 = ⃗휏 dt 훼 total 훼=1 훼=1 If there is no net external torque on system of particles then system’s total angular momentum, L⃗, is constant, dL⃗ if ⃗휏 = 0; then = 0; and L⃗ = constant total dt This is the principle of conservation of angular momentum. True in quantum mechanics as well as in classical mechanics. P. J. Grandinetti Chapter 04: Rotational Motion Orbital and Spin Angular Momentum P. J. Grandinetti Chapter 04: Rotational Motion Orbital and Spin Angular Momentum Consider system of particles again. Total angular momentum relative to origin is ∑N ∑N ⃗ ⃗ ⃗ dr훼 L = l = ⃗r훼 × m훼 i dt 훼=1 훼=1 COM Defining position of each particle relative to center of mass, ⃗ ⃗r훼 = R + r⃗◦훼 ⃗r훼 is position of particle position relative to origin, R⃗ is center of mass relative to origin, O r⃗◦훼 is particle position relative to center of mass. Expression for L⃗ becomes (see notes for derivation) ∑N ⃗ ⃗ ⃗ dr◦훼 L = R × p⃗ + r⃗◦훼 × m훼 «­­¯­­¬total dt 훼=1 relative to origin «­­­­­­­­­¯­­­­­­­­­¬ relative to CM P. J. Grandinetti Chapter 04: Rotational Motion Orbital and Spin Angular Momentum ∑N ⃗ ⃗ ⃗ dr◦훼 L = R × p⃗ + r⃗◦ × m훼 total 훼 dt «­­¯­­¬ 훼=1 relative to origin «­­­­­­­­­¯­­­­­­­­­¬ relative to CM Identify total angular momentum as split into orbital and spin angular momentum terms: ⃗ ⃗ ⃗ L = Lorbital + Lspin ⃗ Imagine Lorbital as angular momentum of Earth as it moves around sun as the origin, ⃗ and Lspin as angular momentum of Earth as it spins about its center of mass. Often true, to a good approximation, that orbital and spin parts are separately conserved. P. J. Grandinetti Chapter 04: Rotational Motion Rotational energy Total kinetic energy of system of particles is 0 1 ∑N 2 1 d⃗r훼 K = m훼 dt 훼=1 2 ⃗ Written in terms of R and r⃗◦훼 gives 0 1 0 1 ⃗ 2 ∑N 2 1 dR 1 dr⃗◦훼 K = M + m훼 2 dt 2 훼 dt «­­­­¯­­­­¬ «­­­­­­­­­­¯­­­­­­­­­­¬=1 center of mass rotation about center of mass 1st term is energy associated with motion of center of mass of system. 2nd term is energy associated with rotational motion of system about center of mass. P. J. Grandinetti Chapter 04: Rotational Motion Rigid Bodies P. J. Grandinetti Chapter 04: Rotational Motion Rigid Bodies Definition When all particles in system are rigidly connected we have a rigid body. This ideal model assumes no relative movement of composite particles. z m5 m2 m3 m1 y m4 x P. J. Grandinetti Chapter 04: Rotational Motion How do we specify the orientation of a rigid body? Definition Euler’s rotation theorem states that orientation of rigid body in a given coordinate system can be described by rotation through an angle about a single axis. Only 2 angles needed to define orientation of rotation axis so full orientation of any rigid body about fixed point can be described by just 3 parameters: e.g., polar, 휃, and azimuthal, 휙, angles and angle of rotation, 휒: Sign of ⃗휒 is determined by right-hand rule: thumb points along rotation axis and right-hand fingers curl in direction of positive rotation. Set of 휙, 휃, and 휒 are called Euler angles P. J. Grandinetti Chapter 04: Rotational Motion Euler angles Another example of a set of Euler angles is convention for giving orientation of an airplane by 3 parameters called yaw, pitch, and roll: Pitch Axis (y) Roll Axis Yaw Axis (x) (z) Yaw, pitch, and roll are just one of many ways of defining the 3 angles implied by Euler’s rotation theorem. P. J. Grandinetti Chapter 04: Rotational Motion Angular velocity vector, ⃗! Definition Angular velocity vector, ⃗!, is vector passing through origin along axis of rotation and whose magnitude equals magnitude of angular velocity, ⃗! ! ⃗ = er ! 휒 ⃗ = d _dt and er is unit vector defined by ⃗ 휃 휙 ⃗ 휃 휙 ⃗ 휃 ⃗ er = sin cos ex + sin sin ey + cos ez Direction of ⃗! is determined by right-hand rule: Curl right-hand fingers in direction of rotation, then thumb points in direction of ⃗!. Keep in mind when describing motion of rigid body that magnitude and orientation of ⃗! can change with time. P. J. Grandinetti Chapter 04: Rotational Motion Angular velocity vector, ⃗! Best origin choice through which ⃗! passes depends on rigid body motion being described. For molecular rotations : natural origin choice is molecule’s center of mass. For top spinning on table surface : origin is better located at fixed point where tip of top meets table surface. z y x y x P. J. Grandinetti Chapter 04: Rotational Motion Relationship between angular momentum and angular velocity vectors Total angular momentum of rigid body relative to origin is ∑N ∑N ⃗ J = .⃗r훼 × m훼v⃗훼/ = m훼.⃗r훼 × v⃗훼/ 훼=1 훼=1 Note: notation change, J⃗ instead of L⃗ for rigid body. th Linear velocity vector, v⃗훼, of 훼 particle is related to its angular velocity, ⃗!, by v⃗훼 = ⃗! × ⃗r훼 ⃗r훼 is particle position. ⃗! is identical for all particles in rotating rigid body since all inter-particle distances are constant. Combining these two expressions ... ∑N [ ] ⃗ J = m훼 ⃗r훼 × . ⃗! × ⃗r훼/ 훼=1 «­­­­­­¯­­­­­­¬ vector triple product P. J. Grandinetti Chapter 04: Rotational Motion Relationship between angular momentum and angular velocity vectors Total angular momentum of rigid body relative to the origin is ∑N [ ] ⃗ J = m훼 ⃗r훼 × . ⃗! × ⃗r훼/ 훼=1 «­­­­­­¯­­­­­­¬ vector triple product Vector triple product has a well known expansion ( ) a⃗ × b⃗ × c⃗ = .a⃗ ⋅ c⃗/ b⃗ *.a⃗ ⋅ b⃗/ c⃗ With triple product expansion we get ∑N [( ) ( ) ] ⃗ J = m훼 ⃗r훼 ⋅ ⃗r훼 ⃗! * ⃗r훼 ⋅ ⃗! ⃗r훼 훼=1 P. J. Grandinetti Chapter 04: Rotational Motion Relationship between angular momentum and angular velocity vectors Moment of Inertia Tensor ∑N [( ) ( ) ] ⃗ J = m훼 ⃗r훼 ⋅ ⃗r훼 ⃗! * ⃗r훼 ⋅ ⃗! ⃗r훼 훼=1 can be rewritten as matrix equation ` a ` a ` ! a Jx Ixx Ixy Ixz x ⃗ r s r s r ! s ⋅ ⃗! J = r Jy s = r Iyx Iyy Iyz s r y s = I ! p Jz q p Izx Izy Izz q p z q «­­­­­­­­­¯­­­­­­­­­¬ «¯¬ I ⃗! matrix I is called the moment of inertia tensor (see next slide). J⃗ = I ⋅ ⃗! When rigid body rotates about origin with angular velocity ⃗! the size and direction of body’s angular momentum, J⃗, is determined by its moment of inertia tensor, I. P. J. Grandinetti Chapter 04: Rotational Motion Moment of Inertia Tensor of a rigid body Elements of Moment of Inertia Tensor about the center of mass If rigid body rotates about its center of mass then relevant moment of inertia tensor–relative to center of mass—is calculated according to ∑N ( ) ∑N 2 2 2 2 Ixx = m훼 y훼 + z훼 * M.Y + Z / Ixy = Iyx = * m훼x훼y훼 + MXY 훼=1 훼=1 ∑N ( ) ∑N 2 2 2 2 Iyy = m훼 x훼 + z훼 * M.X + Z / Iyz = Izy = * m훼y훼z훼 + MYZ 훼=1 훼=1 ∑N ( ) ∑N 2 2 2 2 Izz = m훼 x훼 + y훼 * M.X + Y / Ixz = Izx = * m훼x훼z훼 + MXZ 훼=1 훼=1 M is total mass X, Y, Z are coordinates of the center of mass. Notice that off-diagonal elements are symmetric about diagonal, Ixy = Iyx, Ixz = Izx, etc. Tensors with this property are called symmetric tensors. P. J. Grandinetti Chapter 04: Rotational Motion The Principal Axis System of a Moment of Inertia Tensor Off-diagonal elements are symmetric about the diagonal ` Ixx Ixy Ixz a r s I = r Ixy Iyy Iyz s p Ixz Iyz Izz q For any symmetric tensor one can always find an axis system in which it is diagonal. ` Ixx Ixy Ixz a ` Ia 0 0 a PAS 휙, 휃; 휒 ⋅ r s ⋅ T 휙, 휃; 휒 r s I = R.
Details
-
File Typepdf
-
Upload Time-
-
Content LanguagesEnglish
-
Upload UserAnonymous/Not logged-in
-
File Pages72 Page
-
File Size-