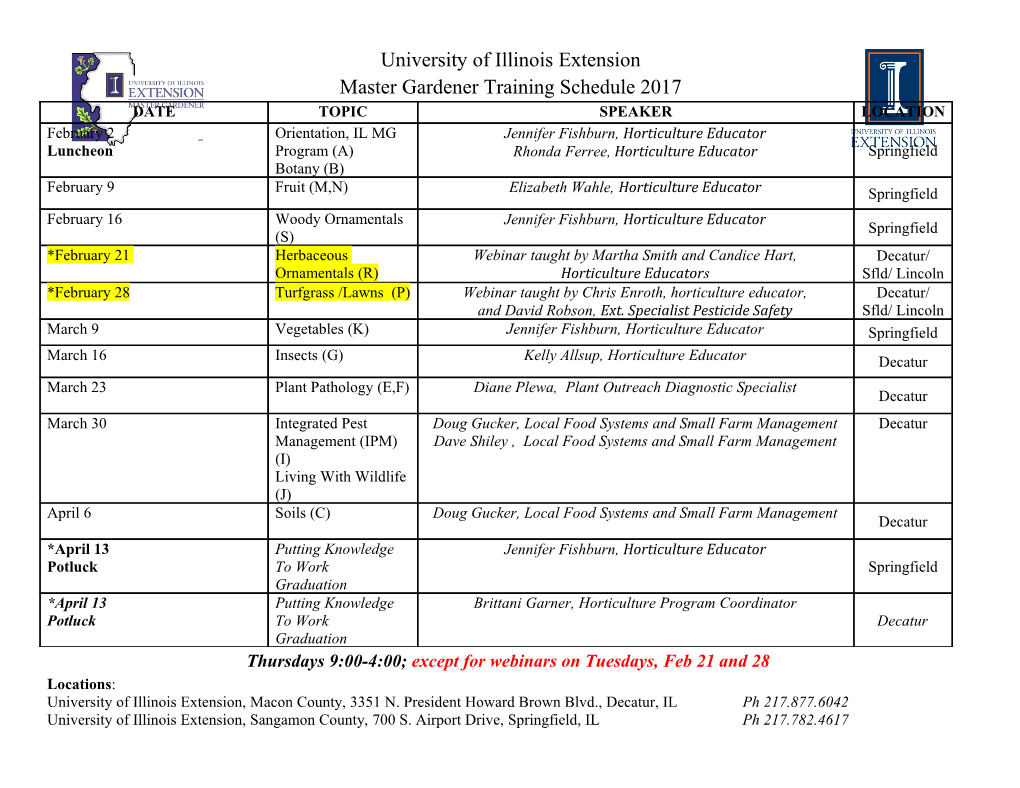
molecules Review Recent Advances in Chemical Biology Using Benzophenones and Diazirines as Radical Precursors 1,2, , 1,2, Muhammad Murtaza Hassan * y and Olasunkanmi O. Olaoye y 1 Department of Chemical and Physical Sciences, University of Toronto Mississauga, 3359 Mississauga Road North, Mississauga, ON L5L 1C6, Canada; [email protected] 2 Department of Chemistry, University of Toronto, 80 St. George Street, Toronto, ON M5S 3H6, Canada * Correspondence: [email protected]; Tel.: +1-905-569-4588 These authors contributed equally to this work. y Academic Editor: Edward Lee-Ruff Received: 18 April 2020; Accepted: 9 May 2020; Published: 13 May 2020 Abstract: The use of light-activated chemical probes to study biological interactions was first discovered in the 1960s, and has since found many applications in studying diseases and gaining deeper insight into various cellular mechanisms involving protein–protein, protein–nucleic acid, protein–ligand (drug, probe), and protein–co-factor interactions, among others. This technique, often referred to as photoaffinity labelling, uses radical precursors that react almost instantaneously to yield spatial and temporal information about the nature of the interaction and the interacting partner(s). This review focuses on the recent advances in chemical biology in the use of benzophenones and diazirines, two of the most commonly known light-activatable radical precursors, with a focus on the last three years, and is intended to provide a solid understanding of their chemical and biological principles and their applications. Keywords: photoaffinity labelling; benzophenone; diazirine; radical precursors; interactome; SABRE; hyperpolarizing agents; crosslinking; photochemistry 1. Introduction The use of radicals or photoactivatable radical precursors has become ubiquitous in the fields of medicinal chemistry and chemical biology in the past three decades. Their main application revolves around studying the interactions of biomolecule targets such as proteins with ligands containing photoactivatable handles. These photoreactive chemical groups (PCG) can be added to a probe, ideally with minimal perturbation of the intrinsic activity of the probe, which is then subsequently introduced into a biological system. After a given incubation period of the photolabelled probe in the biological system, the probe can be activated by light to reveal its biomolecular interactions, thus providing spatial and temporal information. This technique is referred to as photoaffinity labelling (PL). Such applications of chemical systems to study biological systems have transformed the chemical biology field by providing insights into protein interactomes, protein–ligand binding pocket identification, and studying the promiscuity of drugs in biological systems, enabling us to answer the fundamental “what, when, and where” questions of biological interactions. This review focuses on the chemistry of two commonly used PCGs, benzophenone and diazirines, as representatives of two classes of radical precursors (1,2-diradical and carbene radical, respectively; Scheme1), and their applications in biological systems with an emphasis on the last three years. Molecules 2020, 25, 2285; doi:10.3390/molecules25102285 www.mdpi.com/journal/molecules Molecules 2020, 25, 2285 2 of 27 Molecules 2020, 25, x FOR PEER REVIEW 2 of 29 SchemeScheme 1. 1. TwoTwo commonly commonly used radical precursors inin chemicalchemical biology.biology. ((aa)) Benzophenones Benzophenones generate generate a a1,2-diradical 1,2-diradical upon upon irradiation irradiation with with 330–365 330–365 nm nm light. light. ( b(b)) Diazirines Diazirines liberate liberate nitrogen nitrogen and and generate generate a acarbene carbene radical radical upon upon irradiation irradiation with with 340–380 340–380 nm nm light. light. 2. Chemical Principles 2. Chemical Principles 2.1. Benzophenones 2.1. Benzophenones The use of benzophenones (BPs) in PL was first recorded in 1974, when it was shown that they couldThe effi useciently of benzophenones label amino acids (BPs [)1 in]. PL BPs, was like first other recorded ketone in functionalities, 1974, when it was consist shown of a that carbonyl they couldcarbon efficiently that undergoes label amino intersystem acids crossing[1]. BPs, inlike high other yields, ketone making functionalities, it a robust tripletconsist photosensitizer of a carbonyl carbonfor use that in organic undergoes and biologicalintersystem chemistry. crossing Itsin high extensive yields chemical, making and it a physicalrobust triplet properties photosensitizer have been forreviewed use in organic elsewhere and[ biological2] and are chemistry. not the main Its extensive focus of thischemical review; and however, physical notableproperties physical have been and reviewedchemical featureselsewhere pertinent [2] and toare this not review the main are discussedfocus of this herein. review BPs; however, undergo notable two transitions, physical πand–π*, chemicala high-energy features transition, pertinent and to this n– πreview*, a lower-energy are discussed transition. herein. BPs The undergo and n– twoπ* transitiontransitions, occurs π–π*, ata high330–365-energy nm transition, and is well and separated n–π*, a fromlower the-energyπ–π* transition. [The2,3]. and High-energy n–π* transition UV irradiation occurs at has330– a 365tendency nm and to damageis well proteinsseparated due from to the the spectral π–π* overlaptransition of endogenous[2,3]. High-energy fluorophores UV irradiation (e.g., tryptophan, has a tendencytyrosine, histidineto damage etc.); proteins thus, the lower-energydue to the spectral n–π* transition overlap of BPsof endogenous allows them tofluorophores be more applicable (e.g., tryptophan,to biological tyrosine, settings [histidine4]. Although etc.); the thus, n– πthe* transition lower-energy of BPs n– hasπ* transition a lower absorptive of BPs allows spectral them overlap to be morewith biologicalapplicable fluorophores, to biological itsettings has a much [4]. Although weaker absorption the n–π* transition than the π of–π BPs* transition, has a lower as indicated absorptive by spectralits low molar overlap absorptivity with biological coefficient fluorophores, ("max < 300) it [5 ].has This a canmuch be explainedweaker absorption by the poor than orbital the overlap π–π* transition,of the orthogonal as indicated p-orbital by its containing low molar the absorptivity non-bonding coefficient electron (ε lonemax < pair 300) and [5]. πThis* orbital can be reducing explained the byprobability the poor oforbital excitation overlap (Figure of the1). orthogonal The hybridization p-orbital of containing the lone pairs the non on- thebonding carbonyl electron oxygen lone can pair be anddepicted π* orbital in one reducing of two the ways: probability both lone of pairsexcitation are sp (Figure2-hybridized, 1). The hybridization or one lone pair of the is sp-hybridized lone pairs on thewhile carbonyl the other oxygen is an can unhybridized be depicted p-orbital in one of [2, 6two,7]. ways: The non-bonding both lone pairs orbital are containingsp2-hybridized the lone, or one pair loneis depicted pair is belowsp-hybridized as an unhybridized while the other p-orbital is an (Figure unhybridized1)[ 2,6]. Thep-orbital n–π* transition[2,6,7]. The breaks non-bondi the C=ngO orbitaldouble containing bond and generatesthe lone pair a diradical, is depicted which below can as exist an unhybridized in a singlet or p triplet-orbital state (Figure and is1) capable[2,6]. The of ne–ffiπ*ciently transition crosslinking breaks the via C=O covalent double bonding bond and with generates nearby chemo-moieties. a diradical, which The can breaking exist in a of singlet the C= orO tripletdouble state bond and lengthens is capable the bondof efficiently distance, crosslin since theking promotion via covalent of one bonding non-bonding with nearby electron chemo to the- moieties.π* orbital The decreases breaking the of bond the C=O order double of the bond C=O lengthens bond from the 2 tobond 1.5. distance This also, since results the inpromotion a change of in onethe hybridizationnon-bonding electron of the carbon to the atom,π* orbital resulting decreases in a change the bond in theorder geometry of the C=O to a bond non-planar from 2 sp to3 -like1.5. Thispyramidal also results structure in a [ 8change]. in the hybridization of the carbon atom, resulting in a change in the geometry to a non-planar sp3-like pyramidal structure [8]. Excitation of the BP chromophore first yields a diradical in a singlet state (S1), which undergoes Excitation of the BP chromophore first yields a diradical in a singlet state (S1), which undergoes intersystem crossing to quantitatively yield the triplet state (T1). The exact mechanism of the intersystem intersystem crossing to quantitatively yield the triplet state (T1). The exact mechanism of the crossing is controversial [9]. El-Sayed et al. proposed a direct mechanism between the S1 and T1 intersystemexcited states crossing as indicated is controversial by the low-temperature [9]. El-Sayed et Zeeman al. proposed effect a observed direct mechanism in spin alignment between (ZESA) the S1 and T1 excited states as indicated by the low-temperature Zeeman effect observed in spin alignment experiments [8]. Presumably, although the direct S1 to T1 transition violates the El-Sayed selection rules, (ZESA)the BP aromaticexperiments rings [8] lose. Presumably, planarity upon although excitation, the direct allowing S1 to for T1 the transition
Details
-
File Typepdf
-
Upload Time-
-
Content LanguagesEnglish
-
Upload UserAnonymous/Not logged-in
-
File Pages27 Page
-
File Size-