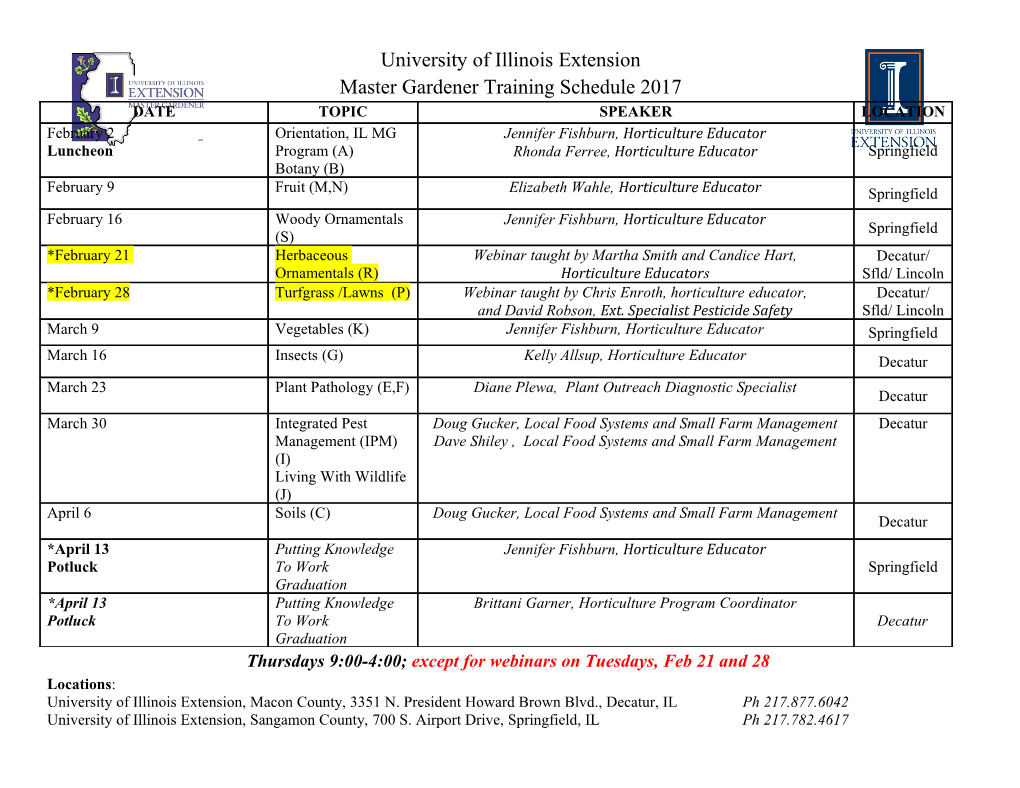
MODELLING THE OB RIVER, IN WESTERN SIBERIA, USING REMOTELY SENSED DIGITAL ELEVATION MODELS S. Biancamaria (1,2) , P. Bates (3) , A. Boone (4) , N. Mognard (1) , J.-F. Crétaux (1) (1) CNES/LEGOS 14 avenue Edouard Belin 31400 TOULOUSE FRANCE Email: [email protected] Email: [email protected] Email: [email protected] (2) Noveltis Parc Technologique du Canal 2 avenue de l'Europe 31520 RAMONVILLE SAINT AGNE, FRANCE (3) School of Geographical Sciences, University of Bristol University Road, BRISTOL. BS8 1SS, UNITED KINGDOM Email: [email protected] (4) CNRM/GAME, Météo-France, CNRS 42 avenue Gaspard Coriolis 31057 TOULOUSE CEDEX, FRANCE Email : [email protected] ABSTRACT Global warming is expected to be most significant in the boreal regions and could greatly affect the discharge regime of arctic rivers. Yet, a modification in the arctic hydrological cycle could then have a feedback on the whole earth climate through increased input of fresh water into the Arctic Ocean. Thus, being able to model major arctic rivers, and therefore predict how they may respond to global warming, is a crucial issue. The aim of this work is to model such an arctic river: the Ob River, in Western Siberia. This has been achieved by coupling the land surface scheme ISBA (Interactions between Soil-Biosphere-Atmosphere) developed by the CNRM (Centre National de Recherche Meteorologique, France) with the flood inundation model LISFLOOD-FP developed by the University of Bristol, UK. Using this coupled macroscale hydrology-hydraulic model with different Digital Elevation Models (DEM) and river depths, the Ob discharge has been simulated and compared to in-situ gauge and satellite data. As expected, the choice of the DEM greatly impacts the simulated inundation extent and therefore the discharge. River depth is also a key parameter to simulate an accurate discharge. It appears that use of a constant river depth between 15 and 20m allows a good simulation of the discharge near the Ob river mouth and this is consistent with the limited available data on Ob river depths. Yet, the flood plain flow is underestimated compared to satellite observation. 1. METHODOLOGY 1.1. Study Domain and time period The study domain corresponds to the Lower Ob River between the cities of Belogorje and Salekhard, which represents roughly the last 900 km of the river before the Ob mouth (Fig. 1). The Ob river is located in Western Siberia, at the east of the Ural Mountain, its drainage basin covers 2,990,000 km 2. By discharge the Ob is the world’s 12th biggest river and the 3rd biggest in the arctic [1]. Its discharge regime is mainly driven by snow melt and rain precipitation between April and September and by rain precipitation from September to November, whereas the river is frozen from November to April. The river thaw occurs very gradually: some parts of the river can still be frozen, whereas in the southest part, the ice thawing can create ice jam which leads to huge inundation. 8 7 6 5 4 3 2 1 Fig. 1. Study domain. The arrows correspond to the lateral inflows from ISBA The aim of this study is therefore to simulate a complex river system in which correct prediction of flow greatly depends on simulating correctly snow accumulation during the winter and the onset of snow melting. For this study we have simulated the whole of 1993 as this year corresponds to the intersection between the time period of the ISBA atmospheric inputs (1982-1994), the available daily discharge measured at Belogorje (January 1993 - October 1994) and the satellite altimetry data (since August 1992 up to now). 1.2. Models Used The river is modelled by the flood inundation model LISFLOOD-FP developed at the University of Bristol [2]. This model predicts water depth in each grid cell at each time step and hence can simulate the dynamic propagation of flood waves over fluvial, coastal and estuarine floodplains. Data requirements are the flood plain topography from a Digital Elevation Model (DEM), the river centreline co-ordinates along with its width and depth, and the Manning coefficients for the river and for the flood plain. The incoming flow to the study domain from the upstream river is given by the daily discharge measured at the Belogorje gauging station. The discharges measured at Belogorje and at Salekhard (which is used to validate the modelled discharge) have been downloaded from the ArcticRIMS website (http://rims.unh.edu). The lateral inflow to the river in the study domain is computed by ISBA (Interactions between the Soil-Biosphere-Atmosphere), which is a Land Surface Scheme (LSS) developed by the CNRM (Centre National de Recherche Meteorlogique) [3]. It has an explicit snow modelling component [4] and can simulate deep soil freeze-thaw cycles [5]. Atmospheric data used as ISBA inputs come from the Global Soil Wetness Project – Phase 2 (GSWP2) forcing data, which were based on ERA-40 re- analysis. The model has been run with the same 1°x1° spatial resolution as ERA-40. A very simple routing model, TRIP [6], has been used to route the runoff from each ISBA pixel to the river. TRIP allows all the pixels from the whole basin to contribute to the lateral inflow. Fig. 2 shows the coupling between ISBA and LISFLOOD-FP to simulate the river discharge and water depth for the river and the flood plain. Incoming flow to the Atmospheric Lateral inflow study domain input (ERA-40) to the river from study domain Discharge from ISBA Belogorje gauging station Runoff from each pixel TRIP Lateral inflow from the whole river basin Flood plain topography LISFLOOD Manning coefficients River centre, (friction) for river and width and depth flood plain River Dischare, water depth and modelling elevation (river & flood plain) Fig. 2. Interaction between the LSS ISBA and the flood inundation model LISFLOOD-FP, with their inputs/outputs. 1.3. River Parameters To model the river, many parameters are needed. A key one is the flood plain topography, which is given by a DEM. For high latitudes very few DEMs are available. The best ones are ACE (Altimeter corrected Elevation) from De Montfort University and GTOPO30 from the USGS (United States Geological Survey). These both have a 30 arc-seconds (~1km) spatial resolution, which is therefore the LISFLOOD output spatial resolution. Yet, after plotting the two DEM (Fig. 3), it becomes obvious that they have artefacts which will greatly affect the simulated flood plain inundation. Indeed, on the study domain below 66°N, ACE has been generated by interpolating ERS-1 data from its geodetic mission. Above 66°N, it uses the same data as GTOPO30. Fig. 3a shows the interpolation artefacts (where the satellite ground tracks can be seen). For GTOPO30, the data come from different Digital Terrain Elevation Data (DTED), with different resolutions and qualities. This is why sometimes there is an obvious offset due to change of data sources, as it is clearly shown by the black dotted circle in Fig. 3b. Some other very important parameters are the river centerline position, width and depth. The river centerline and width have been calculated from the river mask in the CIA World Data Bank II [7], however the river depth is not well known along the river. We have therefore assumed that the bed elevation corresponds to the smoothed DEM elevation along the river center minus a constant river depth. According to [8] maximum depths on the lower Ob can reach 15m to 20m, however to test the impact of uncertainty in this assumption four different values (5m, 10m, 15m and 20m) have been used and simulations run with each of these. Fig. 3. The two DEM available on the study domain: ACE (a.) and GTOPO30 (b.). The black circles highlight significant DEM artefacts. Finally, LISFLOOD-FP needs an estimate of the Manning coefficient for the river and the flood plain. As its value is not well known and to simplify the simulation, typical constant values in space and time have been used equal to 0.03 for the river and 0.06 for the flood plain. 2. RESULTS Fig. 4 and 5 show simulated water elevations for June 08th , 1993 with ACE (a.) and GTOPO30 (b.) DEM data for a 15m and a 10m river depth respectively. Fig. 4c and 5c show, for each pixel, the number of years under water from June 01 st to 08 th for the 2000/2007 time period. This water mask has been computed from MODIS 8-days surface reflectance data. Even though it does not have any information from 1993, it still gives a reasonable estimate of the most frequently inundated area. Artefacts from the DEM, especially for GTOPO30, directly impact the simulated inundation extent. For the 10m and 15m river depth, the flood extent is not well simulated, especially around Salekhard. This observation, which is still true for the 5m and 20m river depths, seems to show that a constant river depth along the lower Ob is not a good approximation: river depths near Salekhard should be lower than river depths near Belogorje. Fig. 4. Water elevation for a 15m river depth with ACE (a.) and GTOPO30 (b.), compared to the water mask from MODIS for the time period 2000/2007 (c.). Fig. 2. Water elevation for a 10m river depth with ACE (a.) and GTOPO30 (b.), compared to the water mask from MODIS for the time period 2000/2007 (c.). After comparing the modelled water extent with the satellite observation, the simulated discharge at Salekhard has been compared to the real measured discharge (Fig.
Details
-
File Typepdf
-
Upload Time-
-
Content LanguagesEnglish
-
Upload UserAnonymous/Not logged-in
-
File Pages8 Page
-
File Size-