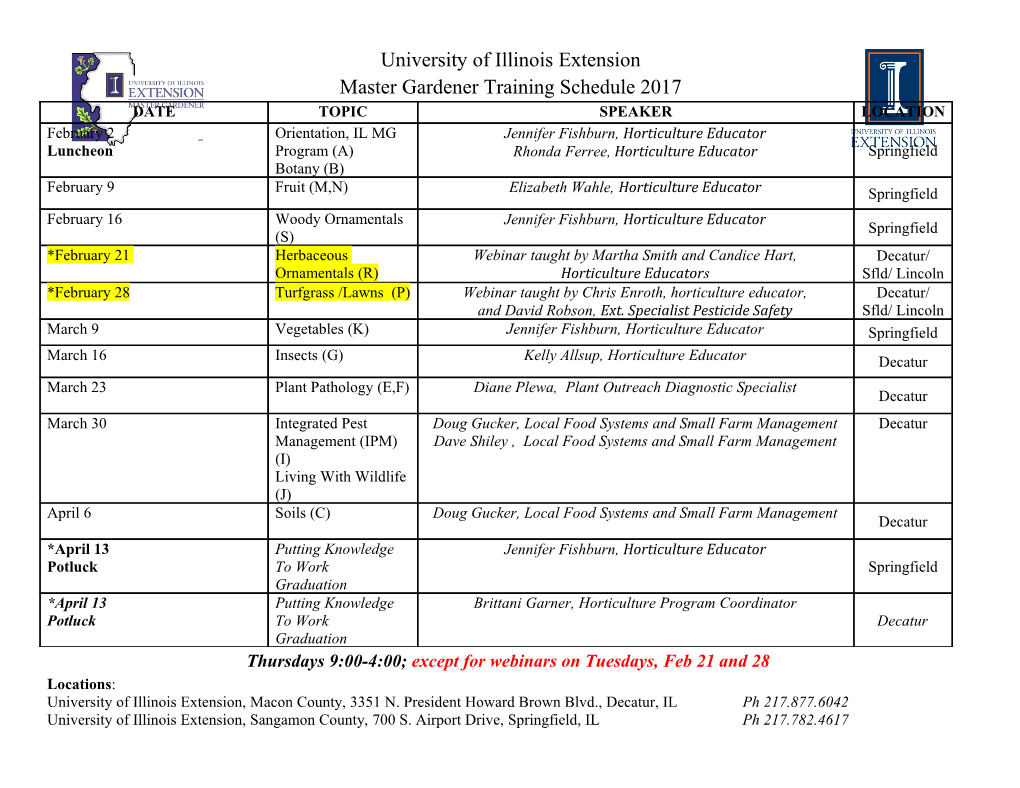
Structure-Strength Correlations in Five Species of Seaweed A Major Qualifying Project Report Submitted to the Faculty of the WORCESTER POLYTECHNIC INSTITUTE In partial fulfillment of the requirements for the Degree of Bachelor of Science in Mechanical Engineering Submitted by: ___________________________________ Rachel Swanson ___________________________________ Rachel Sudol Date: 05/01/2018 Keywords: 1. Seaweed 2. Biopolymers 3. Strength 4. Mechanical Properties 5. Cellulose Approved by: __________________________________ Prof. Satya Shivkumar, Major Advisor Abstract Seaweeds are plentiful and simple to cultivate in most coastal areas of the world. Current industrial uses of seaweed utilize chemically extracted polysaccharides and hydrocolloids found in seaweeds, not entire seaweed fibers. This study investigates the structure and strength of seaweeds found in New England and CA. The samples were analyzed by microscopy, TGA, and tensile testing. Typical breaking forces were between 3 N and 30 N for red and brown seaweed, respectively. Strength for these samples ranged from 0.7 to 29 MPa. The properties of seaweed are determined by the fractions of polysaccharides, cellulose and inorganics. In addition, cellular orientation increases the strength. The organized structure in seaweed and its high strength may enable its use in new biopolymer products. 2 Acknowledgements The authors of this MQP would like to thank Professor Pamela J. Weathers for her aid in helping identify various species of seaweed. We would also like to thank Professor JoAnn L. Whitefleet- Smith for her aid in microscopy as well as Professor Tiffany Butler who helped us with testing our samples. We thank Daryl Johnson for teaching us the TGA machine in the Gateway lab. Finally, we would like to thank Professor Satya Shivkumar for his guidance throughout this process as our advisor. This project would not be complete without him. 3 Table of Contents 1. List of Figures 5 2. Introduction 6 3. Literature Review 8 3.1 Seaweed Structure 8 3.1.1 Morphology 8 3.1.2 Composition 9 3.1.3 Effects of Ecology on Structure 11 3.2 Material Properties and Uses of Seaweed 12 3.2.1 Brown Seaweed 12 3.2.2 Green Seaweed 13 3.2.3 Red Seaweed 13 3.2.4 Carrageenan and Agar Films 13 3.3 Seaweed Biomaterials 14 4. Objectives 15 5. Methods 16 6. Modeling of Tensile Properties 20 7. Results 24 8. Conclusions 27 9. References 29 10. Journal Article 31 11. Appendix 47 4 1. List of Figures Figure 1: Morphology of Seaweed 8 Table 1: Various Components of Seaweed 10 Figure 2: Cellulose Degradation 10 Figure 3: Tensile Strength versus Percent Composition of Cellulose 11 Figure 4: Impact of Temperature on Cell Wall Thickness 12 Figure 5: Seaweed Pressing Method 17 Figure 6: Sample Loaded in Instron Tensile Testing Machine 18 Figure 7: Netzsch TGA Machine and TGA Tested Sample 19 Figure 8: SOLIDWORKS Description of Seaweed Structure: Small Fibers 20 Figure 9: SOLIDWORKS Description of Seaweed Structure: Medium Fibers 21 Figure 10: SOLIDWORKS Description of Seaweed Structure: Large Fibers 21 Figure 11: Small Fiber Tensile Test Simulation 22 Figure 12: Medium Fiber Tensile Test Simulation 22 Figure 13: Large Fiber Tensile Test Simulation 23 Figure 14: Representative Force-Displacement for Each Species of Seaweed 25 5 2. Introduction Seaweed can grow in most coastal areas of the world and needs very little input and care during its growth cycle. This makes it a cheap and widely accessible base for many different applications. It has recently gained attention for its potential to be used to manufacture materials, specifically plastics. These plastics are generally made using solely the polysaccharides in the seaweed, neglecting the mechanical strength that could be gained by using the entire fiber structure of the seaweed plant. Red seaweed, in the phylum Rhodophyta, grows typically in tropical environments in the intertidal and subtidal zones (40 meters to 250 meters deep) at a depth typically deeper than where green and brown seaweed grow due to red seaweed’s ability to absorb blue light [1]. The color of red seaweed comes from the pigments phycoerythrin and phycocyanin. An important subgroup of red seaweed is the coralline algae which releases calcium carbonate onto the surfaces of their cells. Over 6,500 species of red seaweeds are known, the majority of which are marine species [1]. Brown seaweed, in the phylum Phaeophyta, includes mostly marine species and grows in the tidal zones of colder oceans in the world. It is the largest category of seaweed and is usually brown or yellow-brown in color [2]. This brown color is a result of the xanthophyll pigment, fucoxanthin, which masks the chlorophyll pigments. Brown seaweed typically has a branched and filamentous thallus, kelp being the best known example. Brown seaweed is the only type of algae that have internal tissue differentiation into conducting tissue. There are approximately 1800 known species of brown seaweed [1]. The fiber structure of red seaweed is generally filamentous or made of chains of cells attached by end walls and is usually branched. Cortification (husk) or ‘pit plugs’ can occur. ‘Pit plugs’ are granular protein masses that fill holes created during cell division [2]. Brown seaweed are generally filamentous with branches. Many have air bladders which aid in flotation. Some species can be grown meters in height, forming marine kelp forests [1]. The important polysaccharides in red seaweed are floridoside, cellulose, agar, and carrageenan. Floridoside serves as a food reserve and is made up of alpha-d-glucose and amyopectin. Cellulose consists of Beta-1,4 glucose molecules that form microfibrils which make up the cell wall. Agar is a structural polysaccharide that contains D- and L- galactose molecules which makes up part of the matrix that encases the cellulose in the cell wall. Carrageenan is made of only d-galactose units and also surrounds cellulose in the cell wall [3]. The important polysaccharides in brown seaweed are lamarin, alginic acid, and cellulose. Lamarin consists of 20-25 glucose units and beta-1,3 linked glucean which serve as food reserves. Alginic acid is a beta-1,4 linked d-manunonic acid and alpha-1,4 linked l-guluronic acid that forms alginate when the alginic acid chains form around calcium ions to make a stiff gel. Cellulose functions the same way as in the red algae [3]. Trace amounts of iron (Fe), zinc (Zn), Manganese (Mn), and copper (Cu) have been found in both red and brown seaweed [3]. 6 Currently, seaweed is used in a variety of applications. The main use is as a food product, with two million tons of seaweed processed into one type of food or another [2]. The second major use is mainly for the extraction of the phycocolloids alginate, agar and carrageenan [2]. A typical technique that has been used to utilize seaweed and other biopolymers is to grind the material and incorporate it into commodity and engineering thermoplastics [4]. A composite material was made by adding particles of Posidonia oceanica, a red seaweed, with an average particle size of 250 µm with a biobased high density polyethylene. However, seaweed is hydrophilic and most plastics are generally hydrophobic, which leads to improper mixing and thus poor mechanical properties. Materials have also been made from seaweed using only the polysaccharides that can be extracted from it [4]. The polysaccharides are extracted from seaweed and processed in order to produce a plastic resin in combination with traditional thermoplastics [5]. Wong, et al. [6] developed such a plastic in the form of a film, by adding sago starch, agar and glycerol to a solution and drying it overnight at 50°C. These processes do not exploit the fibers of seaweed, which could be used to increase strength of these plastics. A methodology that utilizes the structural feature of seaweed without the need for a matrix of traditional plastics would be highly beneficial both for sustainability, recycling, and material properties. In order to develop such structures, it is imperative to understand the structure-property relationships of different seaweeds. It is the purpose of this contribution to obtain information in this regard. 7 3. Literature Review 3.1 Seaweed Structure Seaweed structure encompass many different components of the seaweed organism. The relevant components to this study, morphology, composition and effect of ecology on structure, are discussed below. 3.1.1 Morphology Seaweed is made up of the thallus, stipe, floats and holdfast. The thallus, seen in Figure 1, is the large leaf-like structure of the organism. The stipe functions as the stem, connecting thallus and holdfast. The holdfast is the roots of the plant, anchoring it to a substrata. The floats are air filled structures that help the plant to be buoyant. The thallus and stipe are consistent across all types of seaweed. Some species of seaweed do not have floats, or are free-floating, so do not have holdfasts [7]. Figure 1: Morphology of Seaweed [7] Seaweeds tend to grow in filaments, which are chains of cells attached by cell walls. These chains are rarely linear, mostly branching at different points throughout growth. Red seaweeds exhibit “pit plugs”, granular protein masses that fill holes left in cell division and filament branching. Red seaweeds often have cortication, in which some cells form a husk or bark around the rest [8]. 8 Seaweeds sometimes show parenchymatous construction, meaning they are made up of differentiated cells. This type of cell is only seen in brown seaweeds. Further, brown and green seaweeds display plasmodesmata, which are structures that link adjacent cells and suggest some degree of cell-to-cell communication [8]. 3.1.2 Composition Polysaccharides A study in the British Phycological Journal found the main food reserve for green seaweed to be starch.
Details
-
File Typepdf
-
Upload Time-
-
Content LanguagesEnglish
-
Upload UserAnonymous/Not logged-in
-
File Pages47 Page
-
File Size-