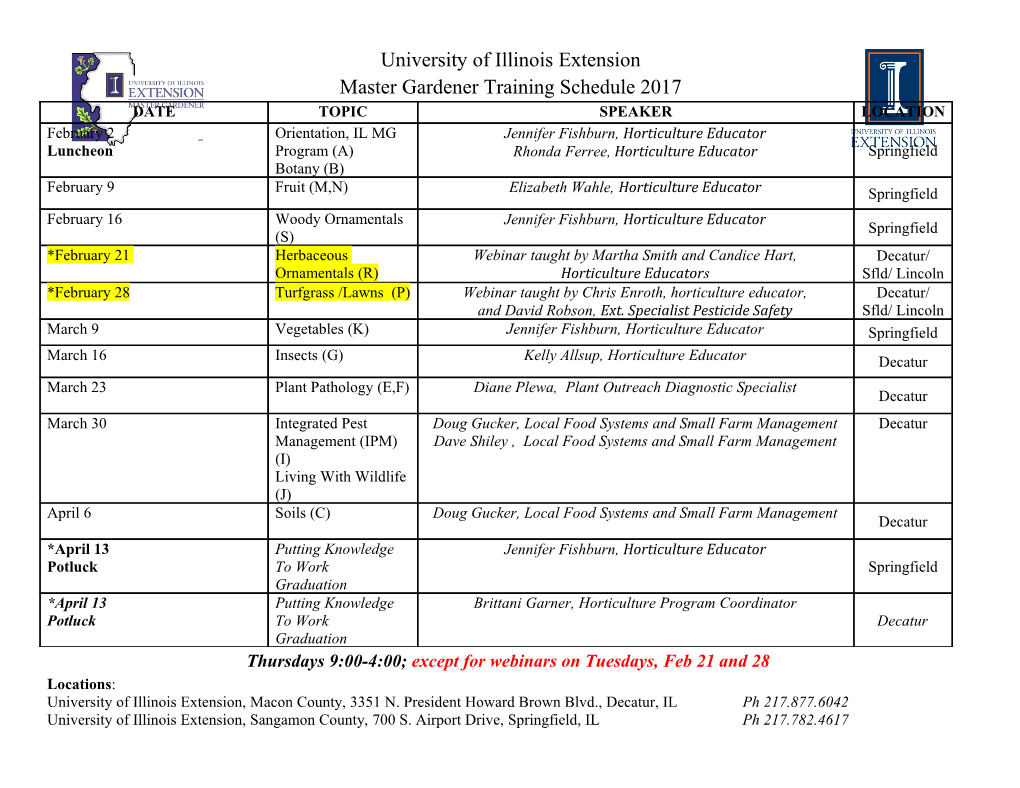
Measuring the Environmental Dependence of Galaxy Haloes with Weak Lensing by Bryan Gillis A thesis presented to the University of Waterloo in fulfilment of the thesis requirement for the degree of Doctor of Philosophy in Physics Waterloo, Ontario, Canada, 2013 c Bryan Gillis 2013 Author's declaration I hereby declare that I am the sole author of this thesis. This is a true copy of the thesis, including any required final revisions, as accepted by my examiners. I understand that my thesis may be made electronically available to the public. ii Abstract We investigate the uses of gravitational lensing for analysing the dark matter haloes around galaxies, comparing galaxies within groups and clusters to those in the field. We consider two cases: when only photometric redshift data is available, and when spectroscopic redshift data is available for a sufficiently large sample of galaxies. For the case of data with photometric redshifts, we analyse the CFHTLenS dataset. This dataset is derived from the CFHTLS-Wide survey, and encompasses 154 deg2 of high-quality shape data. Using the photometric redshifts to estimate local density, we 9 10:5 divide the sample of lens galaxies with stellar masses in the range 10 M to 10 M into those likely to lie in high-density environments (HDE) and those likely to lie in low- density environments (LDE). Through comparison with galaxy catalogues extracted from the Millennium Simulation, we show that the sample of HDE galaxies should primarily (∼ 61%) consist of satellite galaxies in groups, while the sample of LDE galaxies should consist of mostly (∼ 87%) non-satellite (field and central) galaxies. Comparing the lensing signals around samples of HDE and LDE galaxies matched in stellar mass, we show that the subhaloes of HDE galaxies are less massive than those around LDE galaxies by a factor 0:65 ± 0:12, significant at the 2.9σ level. A natural explanation is that the haloes of satellite galaxies are stripped through tidal effects in the group environment. Our results are consistent with a typical tidal truncation radius of ∼ 40 kpc. For the case of data with spectroscopic redshifts, we analyse the GAMA-I and the ongoing GAMA-II surveys. We demonstrate the possibility of detecting tidal stripping of dark matter subhaloes within galaxy groups using weak gravitational lensing. We have run ray-tracing simulations on galaxy catalogues from the Millennium Simulation to generate mock shape catalogues. The ray-tracing catalogues assume a halo model for galaxies and groups, using various models with different distributions of mass between galaxy and group haloes to simulate different stages of group evolution. Using these mock catalogues, we forecast the lensing signals that will be detected around galaxy groups and satellite galaxies, as well as test two different methods for isolating the satellites' lensing signals. A key challenge is to determine the accuracy to which group centres can be identified. We show that with current and ongoing surveys, it will possible to detect 12 15 stripping in groups of mass 10 M {10 M . iii Acknowledgements I acknowledge useful discussions with James Taylor and Hendrik Hildebrant, thank Stefan Hilbert and collaborators for making the results of their ray-tracing simulations available to me, and thank Thomas Erbens, Catherine Heymans, and the rest of the CFTHLenS team for their efforts in processing the CFHTLenS dataset. I would also like to thank my adviser, Mike Hudson, for his guidance and contributions. Based on observations obtained with MegaPrime/MegaCam, a joint project of CFHT and CEA/DAPNIA, at the Canada-France-Hawaii Telescope (CFHT) which is operated by the National Research Council (NRC) of Canada, the Institut National des Sciences de l'Univers of the Centre National de la Recherche Scientifique (CNRS) of France, and the University of Hawaii. This work is based in part on data products produced at TERAPIX and the Canadian Astronomy Data Centre as part of the Canada-France- Hawaii Telescope Legacy Survey, a collaborative project of NRC and CNRS. This work was made possible by the facilities of the Shared Hierarchical Academic Re- search Computing Network (SHARCNET:www.sharcnet.ca) and Compute/Calcul Canada. Funding for SDSS-III has been provided by the Alfred P. Sloan Foundation, the Par- ticipating Institutions, the National Science Foundation, and the U.S. Department of Energy Office of Science. The SDSS-III web site is http://www.sdss3.org/. SDSS-III is managed by the Astrophysical Research Consortium for the Participating In- stitutions of the SDSS-III Collaboration including the University of Arizona, the Brazil- ian Participation Group, Brookhaven National Laboratory, University of Cambridge, Carnegie Mellon University, University of Florida, the French Participation Group, the German Participation Group, Harvard University, the Instituto de Astrofisica de Canarias, the Michigan State/Notre Dame/JINA Participation Group, Johns Hopkins University, Lawrence Berkeley National Laboratory, Max Planck Institute for Astro- physics, Max Planck Institute for Extraterrestrial Physics, New Mexico State University, New York University, Ohio State University, Pennsylvania State University, University of Portsmouth, Princeton University, the Spanish Participation Group, University of Tokyo, University of Utah, Vanderbilt University, University of Virginia, University of Washington, and Yale University. iv Contents Author's declaration ii Abstract iii Acknowledgements iv Contents v List of Figures viii List of Tablesx 1 Introduction1 1.1 Dark Matter Structure.............................4 1.1.1 Structure Formation..........................4 1.1.2 Halo Models..............................7 1.1.3 Halo Substructure...........................8 1.1.3.1 Tidal Stripping of Dark Matter............... 10 1.1.4 Outstanding Issues in LCDM..................... 13 1.1.4.1 Nature of Dark Matter................... 13 1.1.4.2 Missing Satellites Problem................. 14 1.1.4.3 Cusp-Core Problem..................... 16 1.2 Galaxy Formation............................... 16 1.2.1 Cooling................................. 17 1.2.2 Star Formation............................. 20 1.2.3 Stellar Feedback............................ 21 1.2.4 AGN Feedback............................. 23 1.2.5 Environmental Effects......................... 23 1.3 Gravitational Lensing............................. 26 1.3.1 Weak Lensing.............................. 29 1.4 Photometric Redshifts............................. 32 1.5 Group-Finding Methods............................ 34 1.6 Thesis Summary................................ 37 2 Methods Using Photometric Redshifts 39 v 2.1 Observations.................................. 39 2.2 Simulations and Models............................ 41 2.2.1 Simulated Catalogues......................... 41 2.2.2 Models................................. 45 2.2.2.1 Halo Models......................... 45 2.2.2.2 Stripping Models....................... 46 2.2.3 Determining Ellipticity........................ 49 2.3 Catalogue Analysis............................... 51 2.3.1 Determining Environment: The P3 Algorithm............ 51 2.3.2 Galaxy Matching............................ 52 2.3.2.1 Matching Algorithm..................... 52 2.3.2.2 Statistics of Galaxy Selection................ 53 2.3.2.3 Measuring the Lensing Signal................ 56 2.4 Halo Model................................... 57 2.4.1 One-halo term............................. 58 2.4.2 Offset group halo term......................... 58 2.4.3 Underdensity signal.......................... 60 2.5 Fitting Procedure................................ 62 2.6 Results of Photometric Redshift Investigation................ 63 2.6.1 Predictions from Simulations..................... 63 2.6.2 Results from CFHTLenS....................... 65 2.6.3 Alternative Fits............................. 68 2.6.3.1 Truncation Radius...................... 69 2.6.3.2 One-halo Mass and Concentration............. 69 2.6.4 Analysis................................. 69 2.6.4.1 Stellar Mass Biases..................... 70 2.6.4.2 Modeling Inaccuracies.................... 71 3 Methods Using Spectroscopic Redshifts 74 3.1 Simulations................................... 75 3.2 Methodology.................................. 75 3.2.1 Mirror Method............................. 75 3.2.2 Ensemble Method........................... 77 3.3 Analysis of Spectroscopic Redshift Methods................. 77 3.3.1 Dominant Sources of Error...................... 77 3.3.1.1 Shape Noise......................... 78 3.3.1.2 Group Mass and Group Assignment Errors........ 78 3.3.1.3 Centring Errors....................... 79 3.3.2 Lensing Signal around Group Centres................ 81 3.3.3 Lensing Signal around Satellites................... 83 3.3.3.1 Idealized Group Signal Subtraction............ 83 3.3.4 Realistic Group Signal Subtraction.................. 86 3.4 Discussion.................................... 88 3.5 Optimal Methodology............................. 89 3.6 Prospects for Stripping Detections...................... 90 4 Conclusion 93 vi 4.1 Summary of Results.............................. 93 4.2 Implications of Results............................. 95 4.2.1 Strength of Tidal Interactions..................... 95 4.2.2 Colour-Dependence of Stripping................... 97 4.2.3 Modified Gravity Theories....................... 99 4.3 Outlook..................................... 100 4.3.1 Future Surveys............................. 100 4.3.2 Other Lensing Techniques......................
Details
-
File Typepdf
-
Upload Time-
-
Content LanguagesEnglish
-
Upload UserAnonymous/Not logged-in
-
File Pages140 Page
-
File Size-