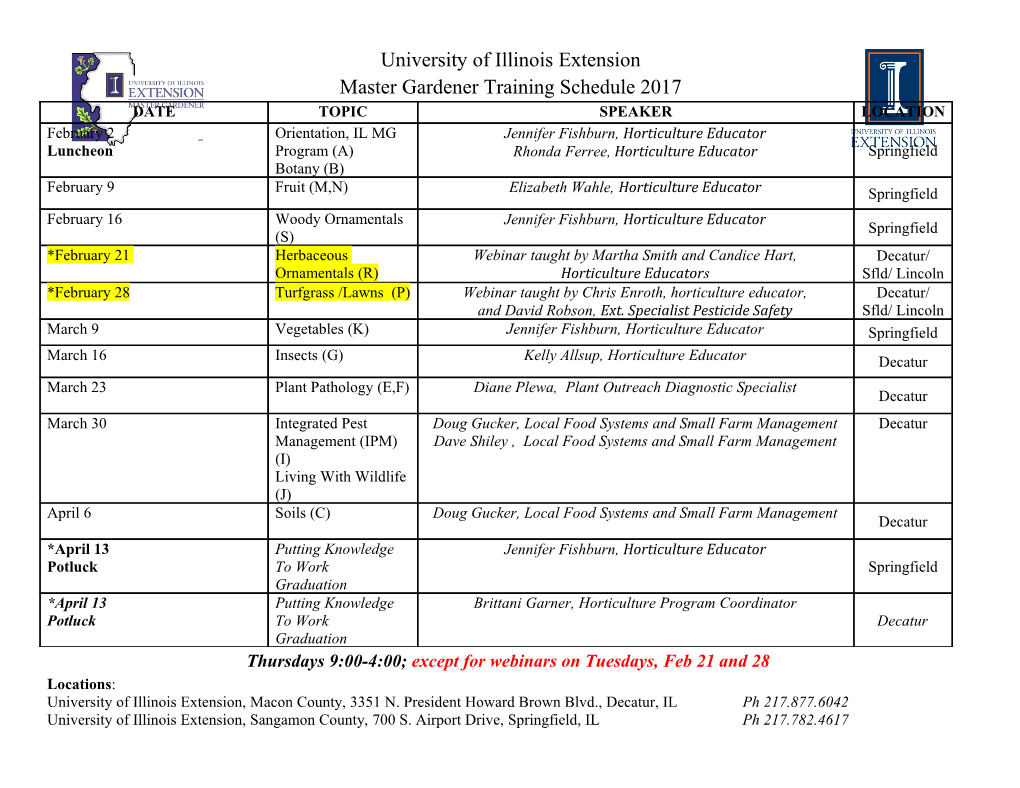
1 Aerodynamics Introduction To be fully in control of your aircraft, pilots need to understand how and why an aircraft flies, as well as the basic physics and mechanics of flight. We call this area of aviation science “Aerodynamics.” This video will go over the basic aspects of aerodynamics that a student pilot needs to understand. This lesson will cover: • The four forces of flight • And the four fundamental maneuvers The Four Forces The study of aerodynamics begins with the understanding of the four forces of flight. While an airplane is in flight, there are four forces acting upon it. They are: lift, weight, thrust and drag. Lift is the upward force, created by the wings as air flows around them; and keeps the airplane in the air. Weight is the downward force toward the center of the Earth, opposite lift, which exists due to gravity. Next, we have thrust. This is the forward force, generally created by the aircraft’s propellers or turbine engines, which pulls or pushes the aircraft through the air. Finally, there is drag. Drag is the force, acting in the direction opposite of thrust, which fundamentally limits the performance of the airplane. When an aircraft is maintaining its heading, altitude, and airspeed, it is said to be in straight-and-level, un-accelerated flight. In un-accelerated flight, lift equals weight and thrust equals drag. Let’s look at these four forces in a little more detail. Lift The key to an aircraft being able to fly is lift. Looking at a cross-section of a wing, we can better understand how the lift gets generated. A wing is a type of airfoil. Airfoils, in general, are just any surface that generates an aerodynamic force as a fluid, in our case AIR, moves around it. Don’t confuse a fluid with a liquid. Fluids are any substance that deform under an applied stress. Liquids, gases, and plasmas are all considered fluids. In addition to the wings, all the flight control surfaces, as well as the propeller are considered airfoils. The aircraft’s fuselage is even an airfoil, but it is not very good at producing lift. Before we get too in depth, let’s introduce a few new terms. The forward-most point of the wing is called the Leading Edge. The aft-most point is called the Trailing Edge. If we connect these two edges together with an imaginary line, this line is called the Chord Line. 2 As an airplane flies through the air, the path that the plane travels along is known as its Flight Path. The airflow that flows around the airplane as it travels through the air is known as the Relative Wind. The Relative Wind is parallel to, but opposite the aircraft’s flight path. The angle between the wing’s chord line, and the aircraft’s relative wind, is called the aircraft’s Angle of Attack. The angle of attack is a major factor as to how much lift the wings generate. So, now that we’ve got those terms out of the way, how does a wing actually create lift? Well, there are two major theories, working in unison, that explain the creation of lift. These are Newton’s Three Laws of Motion, and Bernoulli’s Principle. While all three laws of motion apply to flight, the third law has the most significance to lift production. Newton’s third law states that, for every action, there is an equal and opposite reaction. If you stick your hand out of a moving car’s window, with your palm flat and thumb forward, and then rotate your hand, thumb-side up, you will notice that your hand will want to lift up. By rotating your hand, you are deflecting the air that comes in contact with your hand downward. And, as a result, the air will push your hand up. This is similar to how a wing works. In normal flight, as air flows around the wing the air gets deflected downward, as it flows smoothly around it, and as a result, the wind will lift the wing up. The other main theory of lift is Bernoulli’s Principle. This principle states, “As the velocity of a fluid (in this case, air) increases, its internal pressure decreases.” We can visualize this by having air flow through a tube with a narrower middle section, which we call a venturi. As air enters the tube, it is traveling at a known velocity and pressure. When it arrives at the narrower portion, the velocity increases to allow all the air through. As the air’s velocity increases, the air’s pressure decreases. Then, as the air exits the narrower portion, it returns back to its original velocity and pressure. Now, let’s flatten the top part of the tube. Granted, the effect will not be as pronounced, but there still will be a change in velocity and pressure as the air moves through. Now, how does this relate to a wing? Well, if we replace the bottom protrusion of the tube with a wing, in essence we have the same thing as the venturi. As the air passes over the wing, each layer of air gets deflected less and less, until finally, we reach a layer where the air is not disturbed at all from the wing. This can be thought of as the top of the venturi. An airplane’s wing is shaped similar to that of a venturi. The top is rounded, while the bottom is relatively flat. Because of this, the air traveling over the wing will increase in speed, and as a result, will have a lower pressure than the air below the wing. This imbalance in pressure is called a pressure gradient. Wings are designed to create this kind of pressure gradient because air always moves from areas of high pressure to areas of low pressure. Since the wing is stuck in-between the two areas of unequal pressure, it is “lifted” towards the area of low pressure by the force of the higher pressure air trying to move to the low pressure side of the wing. Now that we’ve covered the two theories behind lift, let’s discuss all the factors that determine how much lift is produced. The best way to discuss this is through the Lift Equation. Don’t worry though; this isn’t a math lesson. 3 Lift equals one half times the air density times the surface area of the wing times the airplane’s velocity squared times the coefficient of lift. For the most part, this should be fairly straight forward. The only one that might confuse you is the coefficient of lift. The coefficient of lift is simply just a number that is associated with a particular shape of an airfoil, as well as the airfoil’s angle of attack. Generally speaking, there are really only two ways a pilot can control the amount of lift the wings can generate: airspeed and angle of attack. The faster the airplane travels, the more lift the wings will generate. Similarly, the higher the airplane’s angle of attack, the more lift the wings will generate. However, there is a limit to this angle. Let’s look at this using a chart. This chart is plotting the coefficient of lift of a particular wing as its angle of attack increases. Lift will continue to increase until a certain angle of attack, called the critical angle of attack. After this point, the wings still create lift, but the amount of lift created is decreased. This is called a stall. When some people think of the word “stall”, they think about the engine stalling. However, we are talking about an aerodynamic stall, which has nothing to do with the engine. Stalls happen when the normally-smooth airflow over the wing separates from the wing’s upper surface, resulting in a turbulent airflow. The parts of the wing that have turbulent airflow passing around them are not producing any lift. For a given airplane, a stall will always occur at the same angle of attack, the critical angle of attack, regardless of its airspeed, attitude, or weight. Here is a wing at a normal cruise angle of attack, something small like 4 or 5 degrees. Increasing the angle of attack will progressively cause more and more disruption of the airflow on the upper surface of the wing. Early on, the airflow will separate at the trailing edge. As the angle of attack increases, the separation of the airflow moves from the trailing edge of the wing to the leading edge. As this separation occurs, the airflow will become more and more turbulent, and less and less lift gets generated. Different airplanes will exhibit different stall characteristics, but generally coincide with mushy, sluggish flight control responses, a stall warning horn, a buffeting or shaking feeling of the airplane and pilots’ controls; and once the stall occurs the aircraft’s nose will pitch down, and the aircraft will begin losing altitude. Since the stall is a result of an excessive angle of attack, the only way to recover from a stall is to reduce the angle of attack below the critical angle. Power should be added to minimize the amount of altitude lost in the recovery and increase the airplane’s speed as quickly as possible. In addition to the aircraft’s airspeed and angle of attack, there are other factors that affect the amount of lift created by the wings. However, the pilot does not have control over these factors.
Details
-
File Typepdf
-
Upload Time-
-
Content LanguagesEnglish
-
Upload UserAnonymous/Not logged-in
-
File Pages10 Page
-
File Size-