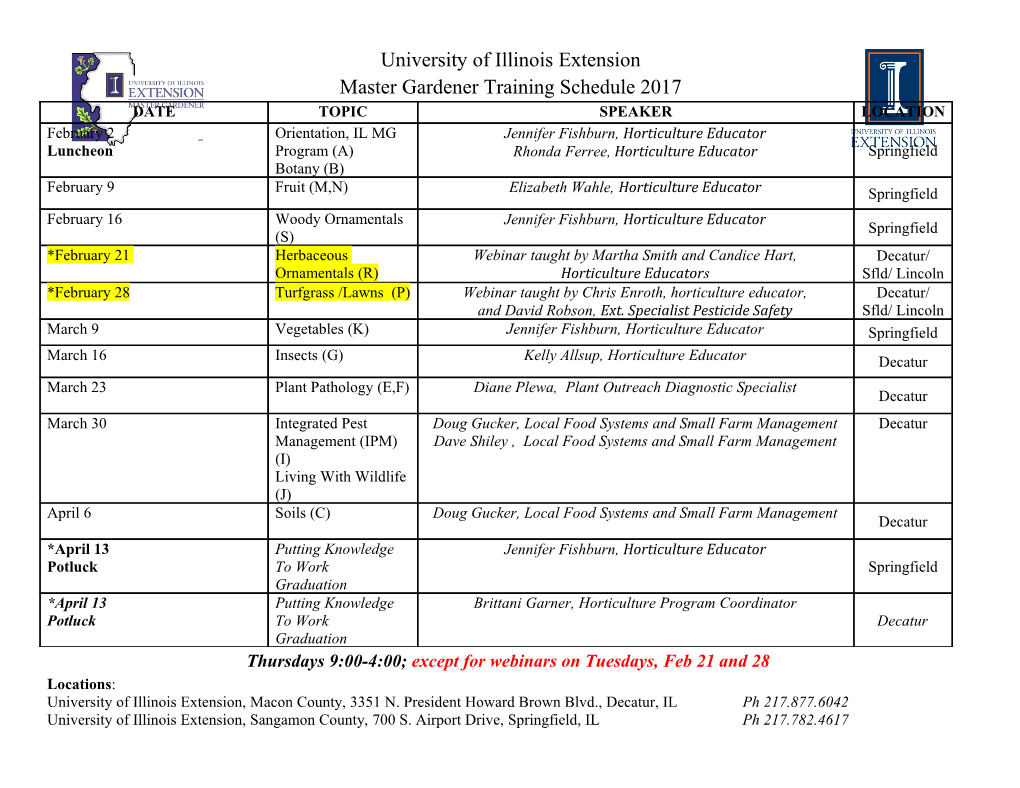
CHAPTER 1 The Measurable Riemann Mapping Theorem 1.1. Conformal structures on Riemann surfaces Throughout, “smooth” will always mean C 1. All surfaces are assumed to be smooth, oriented and without boundary. All diffeomorphisms are assumed to be smooth and orientation-preserving. It will be convenient for our purposes to do local computations involving metrics in the complex-variable notation. Let X be a Riemann surface and z x iy be a holomorphic local coordinate on X. The pair .x; y/ can be thoughtD C of as local coordinates for the underlying smooth surface. In these coordinates, a smooth Riemannian metric has the local form E dx2 2F dx dy G dy2; C C where E; F; G are smooth functions of .x; y/ satisfying E > 0, G > 0 and EG F 2 > 0. The associated inner product on each tangent space is given by @ @ @ @ a b ; c d Eac F .ad bc/ Gbd @x C @y @x C @y D C C C Äc a b ; D d where the symmetric positive definite matrix ÄEF (1.1) D FG represents in the basis @ ; @ . In particular, the length of a tangent vector is f @x @y g given by @ @ p a b Ea2 2F ab Gb2: @x C @y D C C Define two local sections of the complexified cotangent bundle T X C by ˝ dz dx i dy WD C dz dx i dy: N WD 2 1 The Measurable Riemann Mapping Theorem These form a basis for each complexified cotangent space. The local sections @ 1 Â @ @ Ã i @z WD 2 @x @y @ 1 Â @ @ Ã i @z WD 2 @x C @y N of the complexified tangent bundle TX C will form the dual basis at each point. ˝ The inner product on TX extends canonically to a Hermitian product on TX C. @ @ ˝ The matrix of this Hermitian product in the basis @z ; @z is given by f N g (1.2) P P ; O D where 1 Ä1 i (1.3) P : D 2 1 i It follows from (1.1), (1.2) and (1.3) that 1 Ä E GE G 2iF C : O D 4 E G 2iF E G C C Let us introduce the quantities 1 (1.4) .E G pEG F 2/1=2 WD 2 C C 1 E G 2iF (1.5) .E G 2iF / C : WD 4 2 C D E G pEG F 2 C C Note that !1=2 E G 2pEG F 2 (1.6) > 0 and C < 1: j j D E G 2pEG F 2 C C A straightforward computation then shows that " # 1 1 2 2 2 C j j N : O D 2 2 1 2 C j j Since the Hermitian product on TX C is given by ˝ @ @ @ @ ÄC A B ;C D AB N ; @z C @z @z C @z D O D N N N it follows that 2 @ @ 1 2 2 2 2 Á (1.7) A B .1 /. A B / 2 AB 2 A B : @z C @z D 2 C j j j j C j j C N C N N N 1.1 Conformal structures on Riemann surfaces 3 @ @ Now a tangent vector A B TX C is real (i.e., belongs to TX) if and @z C @z 2 ˝ only if B A. This simply followsN from D N @ @ @ @ a b .a ib/ .a ib/ : @x C @y D C @z C @z N Thus, for real tangent vectors, the formula (1.7) reduces to @ @ A A A A : @z C N @z D j C Nj N The last expression suggests that if we are only concerned about lengths of real @ @ tangent vectors, the metric in the complex basis @z ; @z can be represented as f N g (1.8) .z/ dz .z/ dz ; D j C Nj with and defined by (1.4) and (1.5). Let us see how the quantities and associated with transform under a holomorphic change of coordinates z w on X: 7! .z/ dz .z/ dz .w/ dw .w/ dw j C Nj D j C N j .w.z// w0.z/ dz .w.z//w0.z/ dz D j ˇ C Nj ˇ ˇ w .z/ ˇ ˇ 0 ˇ .w.z// w0.z/ ˇdz .w.z// dzˇ ; D j j ˇ C w0.z/ Nˇ from which we obtain .z/ .w.z// w0.z/ D j j w .z/ .z/ .w.z// 0 D w0.z/ or simply .z/ dz .w/ dw j j D j j dz dw (1.9) .z/ N .w/ N : dz D dw dz It follows that .z/ dz and .z/ dzN are well-defined forms on X. Note that z .z/ is a well-definedj j function on X, even though z .z/ is not. 7! j j 7! dz DEFINITION 1.1. .z/ dzN is called the Beltrami form associated with the metric . We say Dis a conformal metric if its Beltrami form is identically zero, in which case .z/ dz is a positive multiple of the Euclidean metric in every local coordinate.D j j 4 1 The Measurable Riemann Mapping Theorem Conformal metrics exist on every Riemann surface. For example, the spherical 2 metric dz =.1 z / on C, the Euclidean metric dz on C, C and the tori j j C j j O j j C=ƒ, and the Poincare´ metric on hyperbolic Riemann surfaces are all conformal metrics. It is clear from (1.8) that two smooth metrics and O have the same Beltrami forms if and only if they belong to the same conformal class, which means =O is a smooth positive function X R. Each conformal class is also called a smooth conformal structure on!X. On the other hand, given a Beltrami dz form .z/ dzN on X, we can pair it with an arbitrary conformal metric .z/ dz Dto construct the metric .z/ dz .z/ dz with the Beltrami formj.j The canonical conformal structureD jof XCis the oneNj represented by any conformal metric .z/ dz on X. It corresponds to the zero Beltrami form 0 which vanishes identicallyj j in every local coordinate on X. COROLLARY 1.2. There is a one-to-one correspondence between smooth dz conformal structures on X and smooth Beltrami forms .z/ dzN which satisfy .z/ < 1 in every local coordinate z on X. D j j Here is a more geometric description for a Beltrami form as a field of concentric ellipses on the tangent bundle of X. Fix a local coordinate z dz D x iy .x; y/ near a point p X and let .z/ N in this coordinate. C Š 2 D dz Consider the family of “circles” E.p/ v TpX v const: which depends only on and not on the choiceD of f the2 representativeW k k D metric.g If v @ @ @ @ D a b .a ib/ .a ib/ , then the “circles” v const: in TpX @x C @y D C @z C @z k k D correspond to the loci .a ib/ .aN ib/ const: in the real .a; b/-plane. i j C C i=2 j D Setting re and .a ib/e , we obtain the loci rN const: in the -plane,WD which is justWD theC family of concentric ellipses withj C the minorj D axis along the real direction and the major axis along the imaginary direction, and with the ratio of the major to minor axis equal to .1 r/=.1 r/ (compare Fig. 1.1). Transferring this family back to the .a; b/-plane,C it follows that E.p/ is a family of concentric ellipses in TpX with 1 angle of elevation of the minor axis arg D 2 1 ratio of the major to minor axis C j j: D 1 j j Note that in this geometric description, the zero Beltrami form 0 corresponds to the field of round circles 2 a2 b2 const: in the .a; b/-plane. j j D C D 1.1 Conformal structures on Riemann surfaces 5 b E.p/ i=2 1=.1 r/ .a ib/e =2 D C a 1=.1 r/ C FIGURE 1.1. Geometric interpretation of a Beltrami form as a field of ellipses on the tangent bundle. To summarize, we have at least three ways of thinking about Beltrami forms on a Riemann surface X: (i) as a . 1; 1/-tensor on X obeying the transformation rule (1.9); (ii) as a conformal structure on X; (iii) as a field of concentric ellipses on the real tangent bundle TX. Now let X and Y be Riemann surfaces and f X Y be a diffeomorphism. W ! Given a Beltrami form on Y , the pull-back f is defined as the Beltrami form of the pull-back metric f , where is any metric with the Beltrami form . It is easy to see that the definition is independent of the choice of . The pull- back operator on Beltrami forms has all the functorial properties of the similar operator on metrics. For example, if f X Y and g Y Z are given W ! W ! diffeomorphisms and is a Beltrami form on Z, then .g f / f .g/, or in short .g f / f g . ı D ı D ı Let us derive a local formula for the pull-back operator. Express f X Y locally as w f .z/, where z and w are local coordinates on X and YW , and! let D 6 1 The Measurable Riemann Mapping Theorem .w/ dw .w/ dw be a metric on Y .
Details
-
File Typepdf
-
Upload Time-
-
Content LanguagesEnglish
-
Upload UserAnonymous/Not logged-in
-
File Pages30 Page
-
File Size-