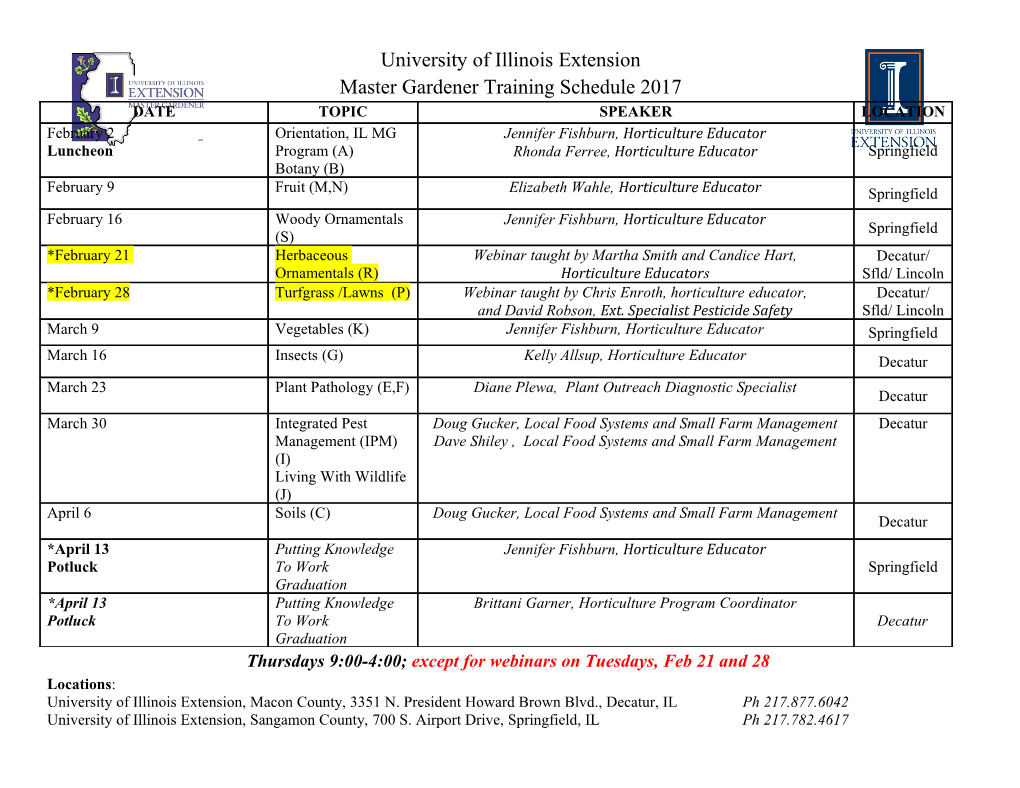
Letter pubs.acs.org/NanoLett Structural Diversity of Arthropod Biophotonic Nanostructures Spans Amphiphilic Phase-Space † ‡ ⊥ ¶ § ∥ ∥ Vinodkumar Saranathan,*, , , , Ainsley E. Seago, Alec Sandy, Suresh Narayanan, # ¶ # ∇ ○ ¶ # ∇ ¶ ◆ ¶ Simon G. J. Mochrie, , Eric R. Dufresne, , , , Hui Cao, , , Chinedum O. Osuji, , ⊥ ¶ and Richard O. Prum*, , † Division of Physics and Applied Physics, School of Physical and Mathematical Sciences, Nanyang Technological University, 21 Nanyang Link, 637371, Singapore ‡ Edward Grey Institute of Field Ornithology, Department of Zoology, University of Oxford, South Parks Road, Oxford, OX1 3PS, United Kingdom § CSIRO Ecosystem Sciences, GPO Box 1700, Canberra, Australian Capital Territory 2601, Australia ∥ Advanced Photon Source, Argonne National Laboratory, Argonne, Illinois 60439, United States ⊥ # ∇ Department of Ecology and Evolutionary Biology, and Peabody Museum of Natural History, Department of Physics, Department ○ ◆ of Applied Physics, Department of Mechanical Engineering and Materials Science, Department of Chemical and Environmental ¶ Engineering, and Center for Research on Interface Structures and Phenomenon (CRISP), Yale University, New Haven, Connecticut 06520, United States *S Supporting Information ABSTRACT: Many organisms, especially arthropods, produce vivid interference colors using diverse mesoscopic (100−350 nm) integumentary biophotonic nanostructures that are increasingly being investigated for technological applications. Despite a century of interest, precise structural knowledge of many biophotonic nanostructures and the mechanisms controlling their development remain tentative, when such knowledge can open novel biomimetic routes to facilely self-assemble tunable, multifunc- tional materials. Here, we use synchrotron small-angle X-ray scattering and electron microscopy to characterize the photonic nanostructure of 140 integumentary scales and setae from ∼127 species of terrestrial arthropods in 85 genera from 5 orders. We report a rich nanostructural diversity, including triply periodic bicontinuous networks, close-packed spheres, inverse columnar, perforated lamellar, and disordered spongelike morphologies, commonly observed as stable phases of amphiphilic surfactants, block copolymer, and lyotropic lipid−water systems. Diverse arthropod lineages appear to have independently evolved to utilize the self-assembly of infolding lipid-bilayer membranes to develop biophotonic nanostructures that span the phase-space of amphiphilic morphologies, but at optical length scales. KEYWORDS: Biophotonic nanostructures, structural colors, iridescence, self-assembly, membrane-folding, biomimetics rganismal colors are commonly produced by pigments In terrestrial arthropods, structural colors are often produced O that selectively absorb specific wavelengths of light and by the well-characterized class of one-dimensional biophotonic 1 re-emit others. However, many organisms also routinely nanostructures comprising thin-film, lamellar (multilayer) produce vivid structural colors by constructive interference of reflectors, or diffraction gratings in the cuticle of the light scattered by mesoscale (100−350 nm) biophotonic 2−4,7 fl 1−4 integument. However, various butter ies (Lepidoptera: nanostructures that are diverse in form and optical function. Lycaenidae, Papilionidae),14,15 weevils (Coleoptera: Curculio- Arthropods, insects in particular, are the most abundant, 7 7 nidae), longhorn beetles (Coleoptera: Cerambycidae), bees diverse, and colorful animals on Earth. Arthropod structural (Hymenoptera: Apidae),16 jumping spiders (Araneomorphae: colors function in a variety of contexts including social and 17 sexual signaling, camouflage, and warning or aposematic Salticidae), and tarantulas (Mygalomorphae: Theraphosi- − 18 communication.5 8 A burgeoning number of studies demon- dae) (see Supporting Information Table S1 for a full list of strate that arthropods are an excellent source of biological inspiration for emerging technologies, such as in sensing, and Received: January 18, 2015 − − photonics2 4,7,9 13 (also see Supporting Information Table S1 Revised: April 30, 2015 for list of references). Published: May 4, 2015 © 2015 American Chemical Society 3735 DOI: 10.1021/acs.nanolett.5b00201 Nano Lett. 2015, 15, 3735−3742 Nano Letters Letter Figure 1. Representative morphology of structural color producing arthropod cuticular nanostructures. (a−f) Light micrographs, (g−l) electron micrographs, and (m−r) representative 2D SAXS patterns from the photonic scales or setae of the following: (a,g,m) Platyaspistes venustus (Curculionidae), single gyroid (I4132) (see Supporting Information Figure S1.105); (b,h,n) Eupholus quintaenia (Curculionidae), single diamond (Fd3m̅) + face-centered orthorhombic (Fddd) (see Supporting Information Figures S1.41 and S1.42); (c,i,o) Sternotomis pulchra (Cerambycidae), simple cubic or single primitive (Pm3m̅) (see Supporting Information Figure S1.130); (d,j,p) Anoplophora graafi (Cerambycidae), quasi-ordered + fcc opal (see Figure 2d and Supporting Information Figures S1.123 and S1.124); (e,k,q) Amegilla cingulata (Apidae), inverse 2D hexagonal columnar (see Figure 2i and Supporting Information Figure S1.137); and (f,l,r) Thyreus nitidulus (Apidae), inverse 2D twisted columnar (see Figure 2h, and Supporting Information S1.139). False color SAXS patterns (unmasked) depict the logarithm of scattering intensity as a function of the scattering wave vector, q. The radii of the concentric circles are given by the peak scattering wave vector (qpk) times the moduli of the assigned hkl indices of permitted Bragg reflections for the assigned space groups. Crystallographic motifs seen in the SEM images are identified in panels g−i. Panel k and its inset respectively show longitudinal SEM, and cross-sectional TEM views of the setae interior. Scale Bars: a, 25 μm; b−f, 50 μm; g,h, 500 nm; i, 250 nm (inset, 100 nm); j, 100 nm; k, 250 nm (inset, 1 μm), l−600 nm (inset, 500 nm); m−r, 0.05 nm−1. Abbreviations: c, chitin; a, air void. references) have structurally colored integumentary scales or addition to one lycaenid butterfly (Lepidoptera: Lycaenidae) setae on their wings, elytra, abdomen, and legs. These and a bee fly (Diptera: Asiloidea: Bombyliidae) (Supporting trichogen-based scales and setae possess a variety of complex, Information Table S1). three-dimensional biophotonic nanostructures composed of the polysaccharide chitin, cuticular proteins, and air (reviewed in ■ RESULTS − refs 2 4, 7, and 15). Despite the growing body of research on We find no evidence of a coherently scattering nanostructure arthropod structural colors (Supporting Information Table S1), present in 19 out of the 140 distinctly colored integumentary we currently lack accurate structural characterizations of more patches (Supporting Information Figure S1 and Table S1). The than a few species. Although knowledge of the development of 14,19−21 SAXS patterns from the rest of the arthropod scales and setae biophotonic crystals in arthropods is growing, we still examined ranged from a large number of discrete Bragg spots in lack a comparative developmental framework to understand the the azimuthal directions (Figure 1m−o,q and Supporting rich biodiversity of arthropod cuticular biophotonic nanostruc- Information Figure S1), characteristic of a polycrystalline tures (discussed in refs 14 and 15) or how these biological nanostructure, to a series of concentric powder-like (or signals function and evolve in these organisms. These complex Debye−Scherrer) diffraction rings (Figure 1p,r and Supporting nanostructures are too large to be developing via classical Information Figure S1), characteristic of an amorphous or morphogenesis as seen in molecular/cell biology, and much too quasi-ordered nanostructure with only short-range isotropic small to be understood using a multicellular developmental order.14,27,28 The presence of a large number of sharp, higher- 14 biology framework. They are also of broader biomimetic order diffraction spots up to 8 or 9 orders from some weevil interest, because synthetic fabrication of three-dimensional and longhorn beetle scales (Figures 1m−o, 2a−c, and photonic nanostructures at these rather large optical length Supporting Information Figure S1) underscores a high degree 22−25 scales remains challenging. of spatial order for a biological system. However, like SAXS Recently, we applied synchrotron small-angle X-ray scatter- experiments on synthetic soft materials,27 40 of the 121 cases ing (SAXS) to identify single gyroid (I4132) photonic crystals exhibited SAXS spectra with variable or ambiguous features that 14 in wing scales of five well-studied butterflies from two families were intermediate between the idealized predictions of and single diamond (Fd3m̅) photonic crystals in the cover crystalline structures. This could be due to both variation in scales of a fossil weevil Hypera diversipunctata.26 Here, we apply length scale of peak structural correlations due to finite long- SAXS to structurally characterize the biophotonic nanostruc- range ordering, which may broaden and flatten diagnostic tures present within scales and setae from 140 distinctly colored spectral peaks, or owing to variation in nanostructure between integumentary patches of diverse land arthropod taxa with different scales of the same species or even different domains prominent structural coloration. We supplemented our SAXS withinasinglespecies.Forthesecases,wepresenta assays with electron microscopy (EM) for 44 out of the
Details
-
File Typepdf
-
Upload Time-
-
Content LanguagesEnglish
-
Upload UserAnonymous/Not logged-in
-
File Pages8 Page
-
File Size-