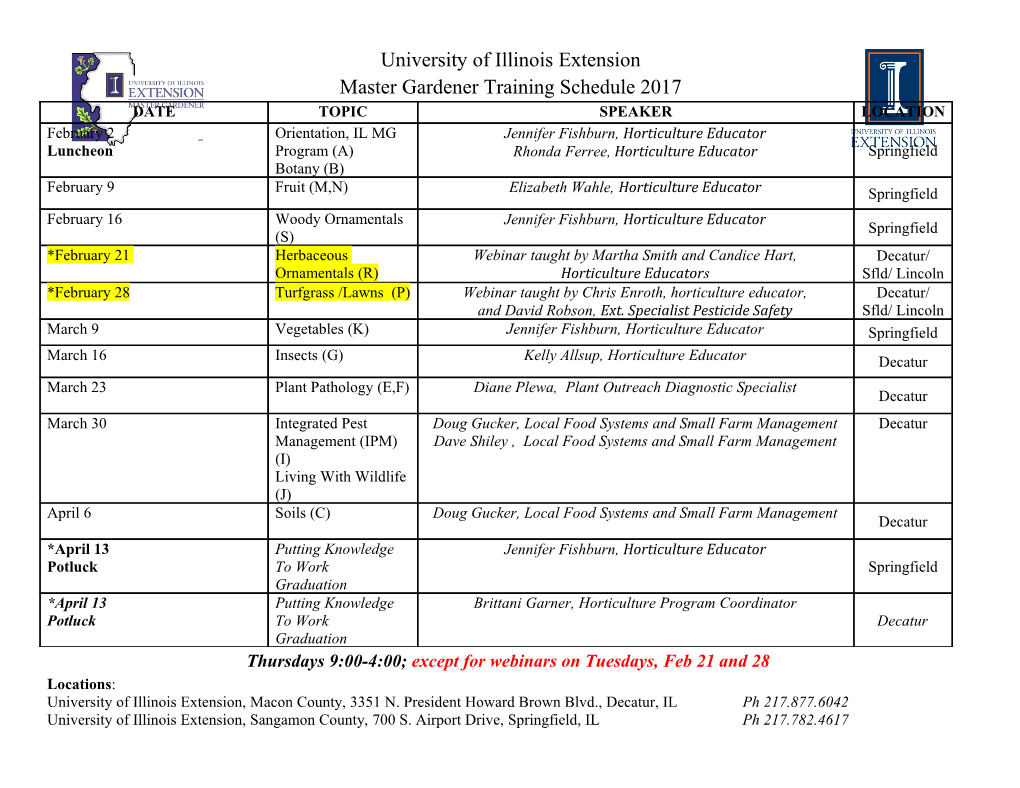
ARTICLE Received 25 Jun 2013 | Accepted 11 Dec 2013 | Published 21 Jan 2014 DOI: 10.1038/ncomms4089 OPEN A source of antihydrogen for in-flight hyperfine spectroscopy N. Kuroda1, S. Ulmer2, D.J. Murtagh3, S. Van Gorp3, Y. Nagata3, M. Diermaier4, S. Federmann5, M. Leali6,7, C. Malbrunot4,w, V. Mascagna6,7, O. Massiczek4, K. Michishio8, T. Mizutani1, A. Mohri3, H. Nagahama1, M. Ohtsuka1, B. Radics3, S. Sakurai9, C. Sauerzopf4, K. Suzuki4, M. Tajima1, H.A. Torii1, L. Venturelli6,7, B. Wu¨nschek4, J. Zmeskal4, N. Zurlo6, H. Higaki9, Y. Kanai3, E. Lodi Rizzini6,7, Y. Nagashima8, Y. Matsuda1, E. Widmann4 & Y. Yamazaki1,3 Antihydrogen, a positron bound to an antiproton, is the simplest antiatom. Its counterpart— hydrogen—is one of the most precisely investigated and best understood systems in physics research. High-resolution comparisons of both systems provide sensitive tests of CPT symmetry, which is the most fundamental symmetry in the Standard Model of elementary particle physics. Any measured difference would point to CPT violation and thus to new physics. Here we report the development of an antihydrogen source using a cusp trap for in-flight spectroscopy. A total of 80 antihydrogen atoms are unambiguously detected 2.7 m downstream of the production region, where perturbing residual magnetic fields are small. This is a major step towards precision spectroscopy of the ground-state hyperfine splitting of antihydrogen using Rabi-like beam spectroscopy. 1 Institute of Physics, Graduate School of Arts and Sciences, University of Tokyo, Tokyo 153-8902, Japan. 2 Ulmer Initiative Research Unit, RIKEN, Saitama 351-0198, Japan. 3 Atomic Physics Laboratory, RIKEN, Saitama 351-0198, Japan. 4 Stefan-Meyer-Institut fu¨r Subatomare Physik, O¨ sterreichische Akademie der Wissenschaften, Wien 1090, Austria. 5 CERN, Gene`ve 1211, Switzerland. 6 Dipartimento di Ingegneria dell’Informazione, Universita` di Brescia, Brescia 25133, Italy. 7 Istituto Nazionale di Fisica Nucleare, Gruppo Collegato di Brescia, Brescia 25133, Italy. 8 Department of Physics, Tokyo University of Science, Tokyo 162-8601, Japan. 9 Graduate School of Advanced Sciences of Matter, Hiroshima University, Hiroshima 739-8530, Japan. w Present address: CERN, Gene`ve 1211, Switzerland. Correspondence and requests for materials should be addressed to N.K. (email: [email protected]). NATURE COMMUNICATIONS | 5:3089 | DOI: 10.1038/ncomms4089 | www.nature.com/naturecommunications 1 & 2014 Macmillan Publishers Limited. All rights reserved. ARTICLE NATURE COMMUNICATIONS | DOI: 10.1038/ncomms4089 ithin the Standard Model of particle physics, CPT to the direct product of mp and me þ . The Zemach and nuclear symmetry is conserved and the spectroscopic proper- polarizability corrections lead to a deviation from the first-order Wties of antihydrogen (H) and its counterpart hydrogen calculation at a level of B40 p.p.m.13 Combined with independent are predicted to be identical. Thus, precise comparisons between measurements of the proton and antiproton magnetic hydrogen and its antimatter counterpart provide sensitive tests moments14,15, the precise determination of the GS-HFS provides of CPT invariance. Historically, the high-precision spectroscopy constraints on CPT symmetry of the electric and magnetic form of hydrogen inspired the development of numerous elegant factors of the two particles. This constitutes an additional CPT experimental methods. This culminated in frequency-comb- symmetry test of the internal structure of protons and antiprotons. locked optical two-photon spectroscopy of the 1S–2S transition, This Article describes the development of an antihydrogen where the remarkable precision of 4.2 Â 10 À 15 was achieved1, source using a cusp trap for Rabi-like beam spectroscopy. A corresponding to an absolute frequency resolution of 10 Hz. significant fraction of antihydrogen atoms in quantum states Other experimental efforts focused on precision spectroscopy of below n ¼ 29 are detected at 2.7 m downstream of the production the ground-state hyperfine splitting (GS-HFS) by using a region, where perturbing residual magnetic fields are small. The B hydrogen maser. Here the GS-HFS transition frequency vHFS of antihydrogen number per hour is estimated to be 25. This is an B1.4 GHz was measured with an absolute precision of 1 mHz important milestone towards the planned precision spectroscopy (ref. 2), which corresponds to a relative precision of 7.0 Â 10 À 13. of the GS-HFS of antihydrogen. The goal of our experiment is the precise measurement of the antihydrogen GS-HFS3. In the framework of the so-called 4 Results Standard Model Extension (SME) , which is an extension of the Experimental set-up. To achieve our physics goal, we have Standard Model allowing Lorentz and CPT violations, GS-HFS developed the experimental apparatus shown in Fig. 1. It consists spectroscopy of hydrogen and antihydrogen provides one of the of an ultra-slow antiproton beam source (MUSASHI)16,a most sensitive tests of CPT symmetry. In addition, the SME positron accumulator17, ultra-low energy beam transport lines predicts that while GS-HFS is directly sensitive to the CPT and the cusp trap, which is a superposition of the strong magnetic violating terms, in the case of the 1S–2S transition frequency it is field gradient provided by the superconducting anti-Helmholtz only a second-order effect. coil and an electrostatic potential10. For the preparation of In contrast to the table-top matter experiments, antihydrogen antiproton and positron plasmas Penning–Malmberg type traps spectroscopy requires complex apparatuses for the production of are used. They comprise a homogeneous strong axial magnetic the elusive antimatter atoms. In addition, antihydrogen atoms field and an electrostatic potential. In all cases MRE18 are cannot be confined in a container made out of matter because introduced into the respective magnets for the electrostatic they immediately annihilate. To overcome this difficulty, the manipulation of the trapped plasmas. Two scintillator modules ALPHA and the ATRAP collaborations at the Antiproton are placed on each side of the cusp magnet, which are utilized to Decelerator (AD) of CERN plan spectroscopy of H confined in track pions produced by antiproton annihilation. This pion- B m magnetic traps with an effective potential depth of only 40 eV. tracking detector provides information about the antiproton Recently both collaborations reported the trapping of ground- annihilation position distribution. Downstream of the cusp trap a state H (refs 5–7). Moreover, the ALPHA collaboration observed hyperfine spectrometer line is placed. It consists of a microwave hyperfine transitions induced in trapped antihydrogen atoms8. cavity to induce H spin flips, a superconducting sextupole magnet However, trapping of H requires strong magnetic field gradients, for spin-state analysis and an antihydrogen detector19. In the which broaden the transition line-widths and reduce experiments described in this article, the microwave cavity is not experimental precision. Thus, we adopt an alternative strategy: 9 used and the detector is placed 2.7 m downstream of the H the Rabi-like spectroscopy of antihydrogen GS-HFS , which is production region. based on polarized antiatomic beams. This scheme uses a so- 10,11 Antiprotons at 5.3 MeV from the AD pass a radio-frequency called cusp trap , which is a combination of the magnetic field quadrupole decelerator (RFQD) that reduces their energy to of a unique superconducting anti-Helmholtz coil assembly and 115 keV. Subsequently, the particles transmit through thin multiple ring electrodes (MRE) for the electrostatic manipulation degrader foils (two 90 mgcmÀ 2 biaxially oriented polyethylene of trapped non-neutral plasmas. The magnetic field gradient terephtalate foils)20 and are trapped in the MUSASHI antiproton produced by the cusp magnetic field exerts a force on the trap. The unique scheme of sequential combination of the RFQD antihydrogen atoms. Low-field-seeking states are preferentially and the MUSASHI trap enables us to use 5–50 times more guided along the cusp trap axis, whereas high-field seekers are de- antiprotons (over 106 in number) per AD cycle than other focused10, resulting in a spin-polarized beam. The atoms are then antihydrogen experiments16. In the MUSASHI trap the passed through a microwave cavity, focused by a spin-selector antiprotons are electron-cooled and radially compressed by a (a sextupole magnet) and finally detected by an antihydrogen rotating electric field21. This preparation procedure enables the detector. When the microwave frequency is equal to the hyperfine efficient transfer of an ultra-low energy antiproton beam to the transition frequency, the low-field-seeking states are converted to cusp trap16. high-field-seeking states and de-focused by the sextupole magnet. Positrons are provided from a 22Na source with an activity of In contrast to the spectroscopic techniques based on trapped 0.6 GBq. The particles are moderated by Ne ice grown on a small antihydrogen, antiatoms of several meV energy can be guided to a cone in front of the source22,23. By interaction with a N /CF gas region with a weak magnetic field. This allows in-flight precision 2 4 À 7 12 mixture the positrons are trapped and further thermalized in the spectroscopy at the level of 10 or better . MRE of the positron accumulator. Subsequently, the accumulated While the 1S–2S transition is primarily an electric phenomenon, particles are transferred to the cusp trap. By repeating this the hyperfine structure is predominantly of magnetic origin. It is sequence, typically 3 Â 107 positrons are loaded into the cusp mainly caused by the interaction of the magnetic moments of the magnet in B10 min. positron me þ and the antiproton mp. The ground state splits into two substates: for parallel magnetic moments (F ¼ 0, singlet state) the effective two-body interaction potential is attractive, while Antihydrogen production in the cusp trap. For the repulsive in the other case (F ¼ 1, triplet state). The zero-field production of antihydrogen atoms a nested well configuration is - 24–26 F ¼ 0 F ¼ 1 transition frequency vHFS is first-order proportional employed , as shown in Fig.
Details
-
File Typepdf
-
Upload Time-
-
Content LanguagesEnglish
-
Upload UserAnonymous/Not logged-in
-
File Pages6 Page
-
File Size-