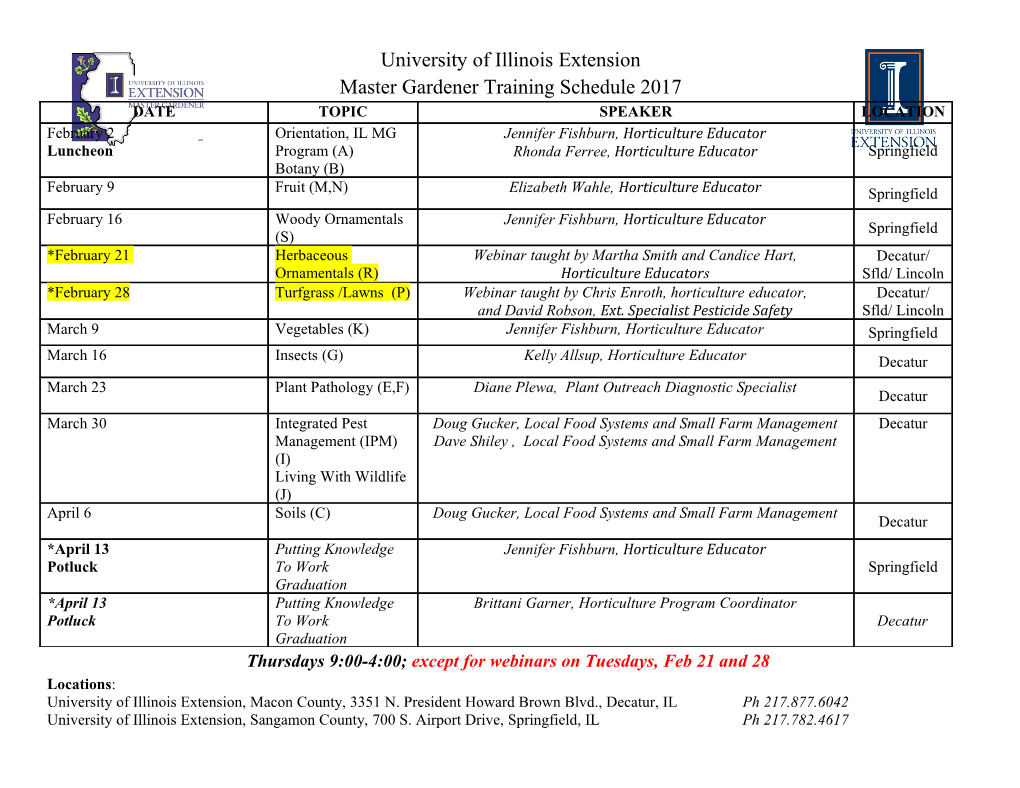
Chapter 25 / Position Effects 357 25 Position Effects Pawel Stankiewicz, MD, PhD CONTENTS BACKGROUND INTRODUCTION CONTACT VS NONCONTACT MODELS OF POSITION EFFECT GENOMIC INSULATORS NONGENIC INTERSPECIES SEQUENCE CONSERVATION CHROMATIN STRUCTURE AND FUNCTION NOVEL METHODS SUMMARY REFERENCES BACKGROUND Position effects describe the observed alteration in protein-coding gene expression that may accompany a change in genomic position of a given gene. A position effect may result from chromosomal translocation or other genomic rearrangements. Recent advances in chromatin studies in several different species including yeast, Drosophila, and mouse have contributed significantly to better understanding of human diseases resulting from abnormal epigenetic effects. Molecular models attempting to explain position effects in humans have been proposed; however, none of them adequately addresses a variety of mechanisms. According to the noncontact models, the cis- or trans-regulatory elements, or locus control regions, are physically separated from the target gene and act either at the RNA level, by protein interactions, or by mediation of boundary elements, termed insulators. On the contrary, the contact models invoke spatial-temporal modifications of chromatin structure (e.g., active chromatin hub). In both models, the conserved nongenic sequences (CNGs) may play an important role in genomic regulation of gene expression. The recent introduction of new techniques including tagging and recovery of associated proteins (RNA-TRAP) and capturing chromatin conformation (CCC or 3C), has provided powerful tools to investigate position effects in humans. INTRODUCTION Chemical modifications to DNA or histones that alter the structure of chromatin without changing the DNA coding sequence are described as epigenetic. Change in histone code, DNA From: Genomic Disorders: The Genomic Basis of Disease Edited by: J. R. Lupski and P. Stankiewicz © Humana Press, Totowa, NJ 357 358 Part V / Functional Aspects of Genome Structure methylation, or genes packaging in the nucleus can result in altered regulation of gene expres- sion that has been referred to as a position effect. Early studies of heterochromatin in Drosophila have led to the discovery of the phenomenon of position effect variegation (PEV), a juxtaposition of a euchromatic gene in a novel cis-acting heterochromatin environment wherein the gene becomes epigenetically silenced (1). Subse- quently, PEV has been described also in yeast (2) and mammals (3–5). The molecular mecha- nisms for PEV have remained elusive, but recent progress in chromatin studies has begun to illuminate molecular aspects of this phenomenon. It has been speculated that the spreading of heterochromatic packaging protects the cell against the invading retrotransposons and trans- posable elements (6). In addition to well known features of heterochromatin, e.g., histone deacetylation, histone methylation (histone H3 on lysine 9, H3-mK9), and heterochromatin- associated protein HP1, small RNAs, RNAi, and siRNAs have been shown in yeast to play important roles in heterochromatin assembly through the RNA-induced initiation of a tran- scriptional gene silencing complex (7–9). The infrequently described trans-regulation is probably best represented in Drosophila by the transvection phenomenon, a pairing-dependent interallelic complementation, or the ability of one locus to affect the expression of the allele, on the homologous chromosome (10). In recent years, transvection has been reported in Drosophila at a rapidly growing number of loci, and transvection-like phenomena have been described in a number of different organisms, including plants, fungi, and mammals (11). Despite the near completion of the human genome sequence, little is known about genomic aspects of gene regulation in humans. Kleinjan and van Heyningen (12) reviewed position effects of chromosomal rearrangements in humans and proposed a few potential mechanisms: (1) separation of the gene from its enhancer or promoter region (locus control region); (2) juxtaposition with an enhancer element from another gene; (3) removal of the long-range insulator or boundary elements; (4) competition with another enhancer; and (5) PEV. A useful model for studying such regulation is provided by genomic rearrangements resulting in dis- eases, in which chromosome breakpoints do not disrupt the causative gene but instead map outside the intact gene. CONTACT VS NONCONTACT MODELS OF POSITION EFFECT Many of the balanced chromosome aberration breakpoints associated with abnormal phe- notype in humans have been mapped up to 400 kb both upstream and downstream from the causative gene (13). Remarkably, a few cases with putative cis-acting regulatory elements have been described as distant as 1 Mb upstream from the target gene (Table 1). Interestingly, no chromosome breakpoints have been found in the genomic regions approx 400–800 kb from the disease-causative gene. This statistically significant bimodal distribution of breakpoints suggests the role of a chromatin structure, rather than the noncontact mechanisms. Preaxial Polydactyly and Sonic Hedgehog SHH Preaxial polydactyly (PPD) (MIM 190605) is one of the most common limb malformation in humans. Belloni et al. (14) and Roessler et al. (15) reported a position effect approx 250 kb upstream of the SHH gene, responsible for PPD. By analyzing a chromosome breakpoint of an apparently balanced reciprocal translocation t(5;7)(q11;q36) in a patient with PPD and a transgenic insertion site in a polydactylous mouse mutant sasquatch (Ssq) (supernumerary Chapter 25 / Position Effects 359 Table 1 Long-Range Position Effects in Humans Maximal distance of breakpoint from gene Gene Rearrangement affected Upstream Downstream type References SOX9 ~900 kb 1.3 Mb Translocation 28,36,37 POU3F4 ~900 kb 111 FKHL7 ~1.2 Mb 112 MAF ~1 Mb 113 SHH ~1 Mb 18 DACH ~1Mb 114 OTX2 115 PLP1 116,117 NSD1 118 preaxial digits of the hind feet) (16), Lettice et al. (17) identified an evolutionary conserved non-coding regulatory element termed ZRS. The ZRS regulatory site is located approx 1-Mb 5' from the sonic hedgehog gene SHH, in intron 5 of the LMBR1 gene. Using a cis-trans genetic test in mice, Lettice et al. (18) and Sagai et al. (19) demonstrated cis- and not trans-interactions between the Ssq locus and the Shh gene. These findings further suggested the presence of a long-range cis-regulating enhancer of SHH, capable of causing congenital abnormalities. Supporting these findings, Lettice et al. (17) identified four different point mutations in the ZRS in unrelated Dutch, Belgian, and Cuban families with PPG that did not have coding mutations in SHH. Further genomic comparison using the computer program VISTA among human, mouse, and teleost fish medaka revealed the mammals-fishes-conserved-sequences MFCS1 (corresponding to ZRS), MFCS2, and MFCS3 in and around LMBR1 (20). Interest- ingly, these elements are located only approx 100 kb from Shh in medaka fish. When deleted in mouse, MCFS1 resulted in a complete loss of Shh expression and truncation of the mouse limb (20). Blepharophimosis and FOXL2 and Aniridia and PAX6 Evolutionarily conserved long-range cis-regulatory elements have been found also in introns of other genes. Three chromosome translocation breakpoints were mapped within introns 6, 11, and 12 of MRPS22 in patients with blepharophimosis/ptosis/epicanthus inversus syndrome (BPES). These breakpoints are located approx 170 kb upstream to the BPES-causitive FOXL2 (21). In addition, four microdeletions with a 126-kb overlap were identified more distal to these breakpoints, 230 kb upstream to FOXL2. Interestingly, the shortest region of deletion overlap contains several CNGs with putative transcription-factor binding sites that represent potential candidate cis-regulatory elements (21a). Moreover, translocation breakpoints have been iden- tified within the last intron of PAXNEB, more than 150 kb downstream of PAX6, the gene responsible for aniridia (22). 360 Part V / Functional Aspects of Genome Structure Campomelic Dysplasia and SOX9 Haploinsufficiency of SOX9 on 17q24.3 causes campomelic dysplasia (CD), (MIM 114290), a clinically distinct syndrome characterized by semilethal skeletal malformation syndrome with or without XY sex reversal (23–26). The two mechanisms responsible for SOX9 haploinsufficiency are intragenic mutations (27) and chromosome rearrangements including translocations, inversions, and deletions (28). The analysis of 12 patients with CD and appar- ently balanced chromosome rearrangements showed the breakpoints scattered up to approx 900 kb upstream of SOX9, whereas the gene itself was intact (29–30). Recently, Pop et al. (31) reported an approx 1.5-Mb microdeletion located approx 380 kb upstream of SOX9. Huang et al. (32) described a chromosome duplication dup(17)(q24.1q24.3) associated with XX female-to-male sex reversal and Bishop et al. (33) reported XX female-to-male sex rever- sal in a transgenic Odd Sex mouse with a 134-kb insertional deletion, resulting from a recom- binant construct insertion 0.98 Mb upstream of SOX9. The authors proposed that a Dct promoter of the inserted construct interacted with gonad-specific enhancer elements, yielding sex rever- sal (34). Thus, both the chondrogenic and gonadal functions of SOX9 appear to be precisely regulated by elements located as far as 1 Mb away from the gene itself. Detailed DNA analysis of the genomic region extending up to 1 Mb proximal to SOX9 failed to uncover any protein-coding genes,
Details
-
File Typepdf
-
Upload Time-
-
Content LanguagesEnglish
-
Upload UserAnonymous/Not logged-in
-
File Pages13 Page
-
File Size-