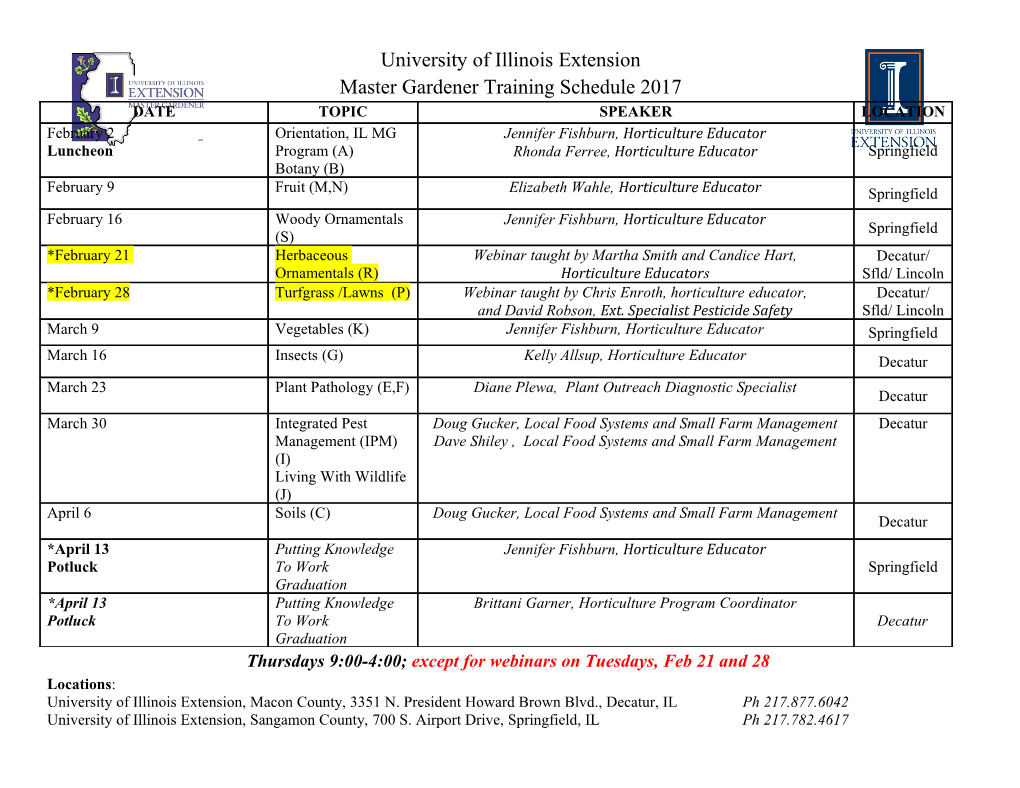
The Cryosphere, 13, 2537–2556, 2019 https://doi.org/10.5194/tc-13-2537-2019 © Author(s) 2019. This work is distributed under the Creative Commons Attribution 4.0 License. Changes of the tropical glaciers throughout Peru between 2000 and 2016 – mass balance and area fluctuations Thorsten Seehaus1, Philipp Malz1, Christian Sommer1, Stefan Lippl1, Alejo Cochachin2, and Matthias Braun1 1Institute of Geography, Friedrich-Alexander-Universität Erlangen-Nuremberg, Wetterkreuz 15, 91058 Erlangen, Germany 2Unidad de Glaciología y Recursos Hídricos (UGRH), Autoridad Nacional del Agua (ANA), 02001 Huaraz, Perú Correspondence: Thorsten Seehaus ([email protected]) Received: 21 December 2018 – Discussion started: 21 February 2019 Revised: 4 July 2019 – Accepted: 5 August 2019 – Published: 30 September 2019 Abstract. Glaciers in tropical regions are very sensitive to for the calibration and validation of glacier change projec- climatic variations and thus strongly affected by climate tions. change. The majority of the tropical glaciers worldwide are located in the Peruvian Andes, which have shown significant ice loss in the last century. Here, we present the first multi- temporal, region-wide survey of geodetic mass balances and 1 Introduction glacier area fluctuations throughout Peru covering the period 2000–2016. Glacier extents are derived from Landsat im- Tropical glaciers in the Peruvian Andes are very sensitive to agery by performing automatic glacier delineation based on climate change and rapidly respond to varying climate set- a combination of the NDSI and band ratio method and final tings (e.g. Kaser and Osmaston, 2002; Rabatel et al., 2013). manual inspection and correction. The mapping of debris- A marked decrease in glacier coverage in Peru has been re- covered glacier extents is supported by synthetic aperture ported by various studies (e.g. Georges, 2004; Hanshaw and radar (SAR) coherence information. A total glacier area loss Bookhagen, 2014; Vuille et al., 2008) for the last decades. of − 548:5 ± 65:7 km2 (−29 %, −34:3 km2 a−1) is obtained The recession of the Peruvian glaciers is proposed to have for the study period. Using interferometric satellite SAR ac- a significant impact on the downstream ecosystem and com- quisitions, bi-temporal geodetic mass balances are derived. munities (Vuille et al., 2018). Glaciers act as an important An average specific mass balance of −296±41 kg m−2 a−1 is temporal water reservoir for precipitation during the wet found throughout Peru for the period 2000–2016. However, season. Glacier meltwater runoff buffers the water short- there are strong regional and temporal differences in the mass age caused by the low precipitation during the dry season budgets ranging from 45±97 to −752±452 kg m−2 a−1. The (Kaser et al., 2003; Schauwecker et al., 2017). The shrinkage ice loss increased towards the end of the observation period. of glaciers leads to higher meltwater discharge and thus in- Between 2013 and 2016, a retreat of the glacierized area of creases water supply to streamflow. However, glacier runoff −203:8±65:7 km2 (−16 %, −101:9 km2 a−1) is mapped and decreases after the glacier loss reaches a critical transition the average mass budget amounts to −660±178 kg m−2 a−1. point (Pouyaud et al., 2005). It has been suggested that some The glacier changes revealed can be attributed to changes in watersheds in the Cordillera Blanca have already crossed this the climatic settings in the study region, derived from ERA- critical transition point (Baraer et al., 2012). An unsteady Interim reanalysis data and the Oceanic Nino Index. The in- or unreliable water runoff is known to cause several socio- tense El Niño activities in 2015/16 are most likely the trig- economic issues (Drenkhan et al., 2019). Hydropower pro- ger for the increased change rates in the time interval 2013– duction and mining rely on a continuous water supply. More- 2016. Our observations provide fundamental information on over, glacier runoff is an important water resource for ir- the current dramatic glacier changes for local authorities and rigation and has the potential to affect large-scale but also subsistence agriculture (Vuille et al., 2018). It also impacts the Andean ecosystems. For example, the bofedales (high- Published by Copernicus Publications on behalf of the European Geosciences Union. 2538 T. Seehaus et al.: Changes of the tropical glaciers throughout Peru between 2000 and 2016 altitude wetlands in the Andes) are very sensitive to changes elled surface mass balance of −1550 ± 620 kg m−2 a−1 for in glacier runoff and a depletion of the meltwater is likely the Andes north of 27◦ S in the period 1979–2014 (Mernild to cause them to shrink (Polk et al., 2017). Additionally, the et al., 2017), an upscaled mass balance of −2 ± 2 Gt a−1 glacier retreat leads to the formation and extension of pro- (−1030 ± 830 kg m2 a−1) for the same region using glacio- glacial lakes (Hanshaw and Bookhagen, 2014; Lopez et al., logical and geodetic mass balances between 2006 and 2016 2010) threatening downstream areas due to their potential to (Zemp et al., 2019), a mass balance calculation throughout cause glacier lake outburst floods (GLOFs). In the Cordillera South America (excluding Patagonia) of −6 ± 12 Gt a−1 us- Blanca, several GLOFs have harmed local communities in ing space-borne gravimetric measurements from the Gravity the past. The most dramatic was the disaster in 1941 when Recovery and Climate Experiment (GRACE) for the period large parts of the city of Huaraz were destroyed by a GLOF 2000–2010 (Jacob et al., 2012), and a geodetic mass budget event, leading to ∼ 1800 casualties (Carey, 2010). However, of −0:49 ± 0:09 Gt a−1 (−227 ± 42 kg m−2 a−1, ice density the possibility of GLOFs is present throughout the tropical scenario: 850 kg m−3) derived from interferometric SAR (In- Andes (Cook et al., 2016; Hoffmann, 2012). SAR) measurements for the period 2000–2012/13 including Several studies have been carried out to map and quantify glaciers in Bolivia (Braun et al., 2019). The first three cover changes in the glacier area in Peru. The majority of the anal- large areas and thus the mass balance signals of glaciers in yses have focused on Peru’s largest glacierized region, the Peru or even smaller regions cannot be derived. The latter Cordillera Blanca (e.g. Baraer et al., 2012; Georges, 2004; uses the glacier boundaries defined by the Randolph Glacier Hastenrath and Ames, 1995; Racoviteanu et al., 2008; Silve- Inventory (RGI) 6.0. The RGI 6.0 has certain limitations in rio and Jaquet, 2005, 2017; Unidad de Glaciologia y Recur- this region (RGI Consortium, 2017), which can lead to biases sos Hidricos, UGRH, 2010), and revealed significant glacier in the mass balance computation (Sect. 6.2). retreat in the last decades. The most recent studies reported a Up to now, a spatially detailed and multi-temporal quan- glacier recession in the Cordillera Blanca of −46 % between tification of glacier changes throughout Peru is missing. In 1930 and 2016 (Silverio and Jaquet, 2017) and −33:5 % be- order to address this issue, this work aims to continue and tween 1975 and 2016 (Veettil, 2018). In other glacierized re- expand the glacier monitoring of previous studies by carry- gions in Peru, distinct glacier retreat was observed as well. ing out a comprehensive analysis of glacier area changes and In the second largest glacierized mountain range in Peru, the mass balances throughout the Peruvian cordilleras for the ob- Cordillera Vilcanota, an area loss of −32 % in the period servation period 2000–2016 based on multi-sensor remote 1985–2006 (Salzmann et al., 2013) and −30 % in the period sensing data. The main objectives of this study are 1988–2010 (Hanshaw and Bookhagen, 2014) was revealed. The only countrywide estimation of glacier area changes was – to obtain a temporally and methodically consistent eval- carried out by the UGRH (2014). They estimated a total uation of countrywide glacier area changes − glacier retreat of 42:64 % from the first Peruvian glacier in- – to assess geodetic glacier mass balances and their tem- ventory of 1970 (Hidrandina SA, 1989) and the recent inven- poral variations throughout Peru tory covering the period 2003–2010 (UGRH, 2014). So far, no multi-temporal nor recent quantification of glacier area – to identify relations between glacier fluctuations, changes throughout Peru is available, only studies at regional changes of climatic variables, and topographic param- levels. eters. There are a few studies dealing with surface elevation and ice volume–mass changes in Peru. Changes in the ice vol- − × −6 3 ume of 57 10 m have been derived from aerial pho- 2 Study site tographs and GPS point measurement data for three glaciers in the Cordillera Blanca by Mark and Seltzer (2005) for Peru is home to the majority of tropical glaciers world- the period 1962–1999. Huh et al. (2017) calculated the wide. About 70 % of all tropical glaciers, covering an area surface elevation changes of six glaciers in the Cordillera of 1602.96 km2 (RGI 6.0), are located there. The Peruvian Blanca by means of photogrammetric digital elevation mod- Andes are subdivided into three major mountain ranges, the els (DEMs) and lidar measurements. They found glacier- Cordilleras Occidental, Central, and Oriental, from west to wide average surface lowering ranging between −9:5 and east, and several smaller cordilleras (Fig. 1). According to −64:06 m in the period 1962–2008. In the Cordillera Vil- Sagredo and Lowell (2012), the glacierized areas are divided canota, Salzmann et al. (2013) estimated volume changes into three subregions based on their climatic settings. of −40 % to −45 % based on inventory parameters for the period 1962–2006.
Details
-
File Typepdf
-
Upload Time-
-
Content LanguagesEnglish
-
Upload UserAnonymous/Not logged-in
-
File Pages20 Page
-
File Size-