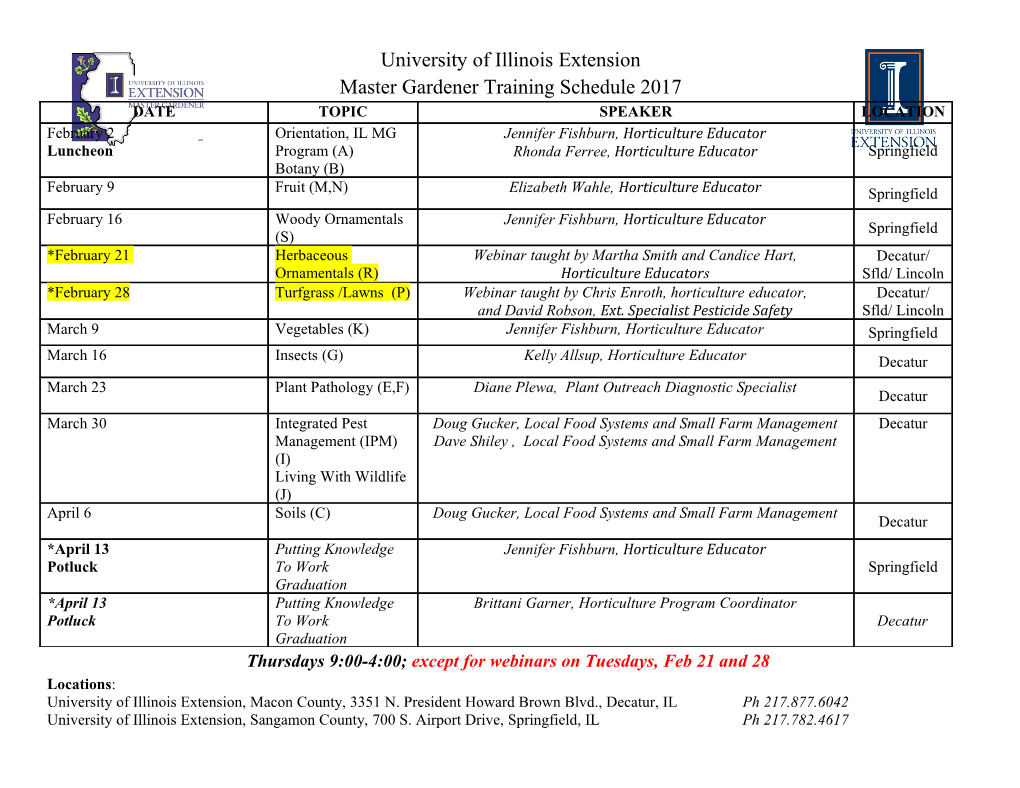
Appendix A Astronomical Imaging Camera technology has developed remarkably quickly over the past two decades. Just as daylight photography has been transformed by the arrival of digital cameras—which are now so small and cheap that they can be embedded into laptops, smartphones and tablet computers—many of the same devices are also ideally suited for astronomical photography. Before their sudden arrival, photo- sensitive films had been the only technology available for astronomical photogra- phy, and had been in use for almost 150 years. In the 1990s, digital cameras were crude devices, ideal for beginners who wanted the instant feedback that their displays could provide, or who liked the ease of not having to develop endless rolls of film. But they could not compete with the high-end film cameras used by more serious photographers, since their sensors lacked the very high resolution that could be reached by the best film cameras. Each pixel in a digital camera is a tiny light-sensitive electronic component, which needs to be wired up. Making these pixels small enough to rival the resolution that could be achieved by the traditional method of spreading light-sensitive chemicals across a film proved very challenging. Since then, however, digital cameras have sold in such vast numbers that it has become economic to put a vast development effort into building sensors with ever- larger numbers of ever-smaller pixels. At the time of writing, cameras are routinely built with more than ten million pixels (megapixels), fabricated and sold at a cost of much less than a thousandth of a cent per pixel. The turning point came in around 2005, when the resolution of moderately-priced digital cameras had become high enough that even most professional photographers found their images no longer inferior to those produced by film cameras, but their instant feedback much more convenient to use. D. Ford, The Observer’s Guide to Planetary Motion: Explaining the Cycles of the Night Sky, 215 The Patrick Moore Practical Astronomy Series, DOI 10.1007/978-1-4939-0629-1, © Springer Science+Business Media, LLC 2014 216 A Astronomical Imaging Simultaneous with the revolution that has occurred in daylight photography, astrophotographers have also almost universally hung up their film cameras in favor of digital devices. Digital sensors have turned out to perform substantially better than film in low-light conditions, meaning that the gap between the image quality achieved by terrestrial and astronomical photographers has closed considerably. Even though developing cameras specifically for astronomical use is highly expen- sive, it has been possible to merely repackage chips built for webcams into cases that can be mounted onto the back of a telescope. This is a fast-moving area, and so this appendix does not recommend particular camera models, but it provides an overview of some of the techniques used by astrophotographers which are likely to remain constant in years to come. Historical Astrophotography The art of astrophotography is almost as old as that of photography itself. The nineteenth century polymath John Herschel was not only an astronomer, but also a chemist who experimented with early photographic films, inventing the cyanotype process in 1842. The intrinsic faintness of astronomical objects has always been a challenge for those trying to capture them on film. Indeed, the principal problem that almost all astrophotographers struggled to solve until very recently was to find ever-more sensitive films or sensors, which would reduce exposure times to within reasonable bounds. The first known successful astrophotograph was taken by John Draper (1811–1882) in March 1840, who caught the Moon on film with a 20-min exposure. The improvement in technology that has occurred in the 170 years since then is demonstrated by the fact that a modern astrophotographer would typically use an exposure of around a tenth of a second or less to take the same image today of this, the night sky’s brightest object. By the mid nineteenth century, progress was being made in developing more sensitive films and the range of objects accessible to astrophotographers was grow- ing. In 1850, Vega became the first star to be captured on film, photographed by William Bond (1789–1859) and John Whipple (1822–1891). The following year’s solar eclipse was the first that is known to have been photographed. The first image of the spectrum of a star followed in 1863. Faint fuzzy objects—nebulae—proved considerably more difficult, and the first image of the Orion nebula (M42) was not captured until 1880, by Henry Draper (1837–1882) using a 51-min exposure through an 11-in. refractor. Many of the problems with which the pioneers had to grapple remain current today. Any telescope that is used for astrophotography must remain firmly centered on the same particular patch of sky for the whole duration of each exposure, to avoid forming a blurred image. The telescope must follow the sky’s diurnal rota- tion, and although a motorized drive can help, inevitably any device that simply rotates the telescope in right ascension once every 23 h and 56 min will have defects that cause it to drift over time. Among these, the telescope’s mount may not be A Astronomical Imaging 217 properly aligned to the celestial pole, causing the drive to rotate the telescope about the wrong point; alternatively, the drive motor itself may not move at a perfectly steady rate; or the drive motor may not run at quite the right speed. Moreover, even the sturdiest mount may have some susceptibility to being shaken by the wind. To correct for any or all of the above, a device called a guider—which may be either human or automated—is usually needed to monitor the alignment of long expo- sures and occasionally give the telescope a nudge. Three years after Henry Draper’s initial image of the Orion nebula, Andrew Common (1841–1903) and George Calver (1834–1927) pioneered a new mecha- nism for manually guiding telescopes over long periods, which allowed them to expose a single piece of film for 90 min in 1883 (see Fig. A.1 ) without appreciable drift in the telescope’s pointing. Later in the same decade, the Paris Observatory was confident enough about the new technology to establish a world-wide collab- orative project to photographically survey the whole sky—the Cartes du Ciel— though this over-ambitious project ended up taking decades to complete, and the last observations not taken until 1950. The full survey was eventually published, nearly 80 years later, in 1964. The Advantages of Digital Sensors Despite over a century of refinements to the chemistry of photographic films, digi- tal sensors have brought three immediate advantages over film photography. Firstly, the sensors themselves are intrinsically much more sensitive to light. Even the most sensitive films—often chemically modified by astronomers using a range of tech- niques collectively called hypersensitization—only respond to a few percent of the photons of light that fall on them. By contrast, modern charge-coupled devices (CCDs) typically have quantum efficiencies in excess of 70 %, meaning that more than 70 % of photons falling on them are detected, and correspondingly that expo- sures of a fraction of the length are needed to reveal comparable detail. Secondly, digital sensors have vastly superior dynamic range to film. Many astronomical objects have large contrasts between bright features and fainter struc- tures that are visible around them. This is especially true in the deep sky: the central regions of galaxies and globular clusters often vastly outshine their extremities. But it is also an issue that affects any planetary imager who wants to photograph the Moon occulting Jupiter, Mars passing through the Pleiades, or the moons of Saturn alongside their parent planet. Mars’s two moons Phobos and Deimos are so faint that they are almost impossible to separate from its glare, and even with modern sensors, anyone who manages to capture an image of either can count themselves an astrophotographer of the highest caliber. Photographic film invariably has poor dynamic range, because individual pho- tons are more likely to trigger chemical reactions in the film in the presence of other photons. In other words, well illuminated areas of photographic film are more sensitive to light than less illuminated areas. This effect, known as reciprocity 218 A Astronomical Imaging Fig. A.1 The development of astronomical photography: the Orion nebula (M42), as photo- graphed by Henry Draper (1880; top-left ), Andrew Common and George Calver (1883; top- right ) and by the Hubble Space Telescope ( bottom ) A Astronomical Imaging 219 failure, makes it very easy to take images where bright areas of the frame are over- exposed, while darker areas are simultaneously under-exposed. By contrast, modern CCDs work by counting the number of individual photons that land on each individual detector element (pixel), and so their response is very nearly linear: a pixel which reports twice the illumination of another pixel received twice as many photons during the course of the exposure. Perhaps the greatest revolution brought by digital sensors, however, has been the immediate feedback that they provide. In the past, focusing was a highly approxi- mate art, with no direct means of assessing the sharpness of the image that was falling onto a piece of film until it came to be developed. Effects such as the thermal contraction of a telescope tube over the course of the night due to the falling ambient temperature were virtually impossible to mitigate. The result was that pho- tographic setups gradually drifted in and out of focus. By contrast, it is now possible to routinely take test images throughout the night, and the focal position of a camera can be periodically adjusted.
Details
-
File Typepdf
-
Upload Time-
-
Content LanguagesEnglish
-
Upload UserAnonymous/Not logged-in
-
File Pages23 Page
-
File Size-