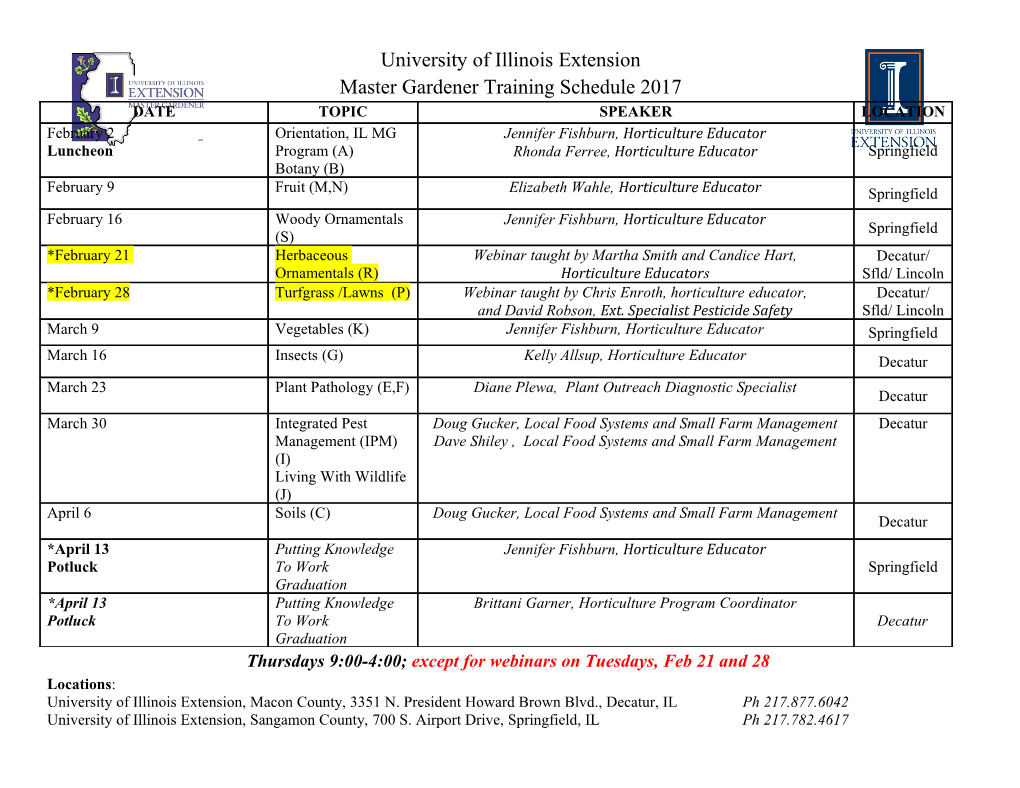
Differential Geometry of Some SURFACES IN 3-SPACE Nicholas Wheeler December 2015 Introduction. Recent correspondence with Ahmed Sebbar concerning the theory of unimodular 3 3 circulant matrices1 × x y z det z x y = x3 + y3 + z3 3xyz = 1 y z x − brought to my attention a surface Σ in R3 which, I was informed, is encountered in work of H. Jonas (1915, 1921) and, because of its form when plotted, is known as “Jonas’ hexenhut” (witch’s hat). I was led by Google from “hexenhut” to a monograph B¨acklund and Darboux Transformations: Geometry and Modern Applications in Soliton Theory, by C. Rogers & W. K. Schief (2002). These are subjects in which I have had longstanding interest, but which I have not thought about for many years. I am inspired by those authors’ splendid book to revisit this subject area. In part one I assemble the tools that play essential roles in the theory of surfaces in R3, and in part two use those tools to develop the properties of some specific surfaces—particularly the pseudosphere, because it was the cradle in which was born the sine-Gordon equation, which a century later became central to the physical theory of solitons.2 part one Concepts & Tools Essential to the Theory of Surfaces in 3-Space Fundamental forms. Relative to a Cartesian frame in R3, surfaces Σ can be described implicitly f(x, y, z) = 0 but for the purposes of differential geometry must be described parametrically x(u, v) r(u, v) = y(u, v) z(u, v) 1 See “Simplest generalization of Pell’s Problem,” (September, 2015). 2 That story is summarized in a companion essay, “Some remarks concerning the sine-Gordon equation,” (November, 2015). 2 Differential geometry of surfaces in 3-space Tangent to Σ at the (non-singular) generic point r are the vectors ru and rv, which are, in general, neither normalized nor orthogonal, but which will be assumed to be not parallel. The local metric structure of Σ is indicated by ds2 = dr dr = (r du + r dv) (r du + r dv) · u v · u v = r r dudu + 2r r dudv + r r dvdv u· u u· v v· v E(u, v)du2 + 2F (u, v)dudv + G(u, v)dv2 (1) ≡ i j 1 2 = gij(u, v)du du : here u = u, u = v where g g E F (u, v) = 11 12 = G g g F G % 21 22 & % & is the familiar metric tensor. Equation (1) is known as the 1st fundamental form. The unit vector r r r r N = u × v = u × v r r r r sin ω | u × v| u v is normal to both ru and rv, and therefore stands normal to Σ at r. Differential variation of N dN = N udu + N vdv provides indication of the local curvature of Σ. That information is folded into the structure of the 2nd fundamental form dr dN = r N dudu + (r N + r N )dudv + r N dvdv − · − u· u u · v v · u v· v which by arguments'of the form ( (r N ) = 0 = r N + r N = r N = r N p· q q p· q pq· ⇒ − p· q pq· can be written dr dN = r N du2 + 2r N dudv + r N dv2 − · uu · uv · vv · e(u, v)du2 + 2f(u, v)dudv + g(u, v)dv2 (2) ≡ i j = hij(u, v)du du with h h e f (u, v) = 11 12 = H h h f g % 21 22 & % & From ds2 > 0 it follows that the metric matrix G must be positive-definite (both eigenvalues positive, their product det G > 0). No such condition pertains, however, to H, the determinant of which can be positive at some points P on Σ, vanish or be negative at other points. We shall, in fact, have special interest in “hyperbolic” surfaces, on which at all points det H < 0.3 3 It is unfortunate that tradition has assigned roles to the symbols G and g to which the conventions of Riemannian geometry have assigned other roles; we are thus precluded from writing (for example) g = det G. I will depart only occasionally from tradition, and endeavor always to minimize the possibility of confusion. Fundamental forms & the Gauss equations 3 G conveys information that is locally intrinsic to the surface. Not so H, which by its N -dependence is rendered extrinsic. The Gauss equations. These present ruu, ruv and rvv as linear combinations of r , r , N . They can be produced as corollaries of the following { u v } lemma: Let a, b, c —linearly independent, but subject to no additional restrictions—comprise{ }an arbitrary basis in 3-space, and let the arbitrary vector x be developed x = αa + βb + γ c Then a a a b a c α a x · · · · b ·a b ·b b ·c β = b ·x · · · · c·a c·b c·c γ c·x · · · · which if c is a unit vector normal to both a and b becomes a a a b 0 α a x · · · b ·a b ·b 0 β = b ·x · · · 0· 0· 1 γ c·x · giving α b b a b 0 a x · · · β = 1 a· b −a a· 0 b ·x D · · · γ −0· 0· D c·x · where a a a b D = (a a)(b b) (a b)2 = det · · · · − · a b b b % · · & We therefore have x = D –1 (b b)(a x) (a b)(b x) a · · − · · + D –1 (a a)(b x) (a b)(a x) b + (c x)c (3) ) · · − · · * · Now set a r , b r , c ) N and look to the case* x r . We then have → u → v → → uu g g D = det 11 12 , which at risk of confusion will be denoted g g g % 21 22 & r = g–1 g (r r ) g (r r ) r + g–1 g (r r ) g (r r ) r uu 22 u · uu − 12 v · uu u 11 v · uu − 12 u · uu v + (N r )N ) * ) · uu * which by g11 g12 g g = g–1 22 21 g21 g22 g −g % & % − 12 11 & becomes r = g11(r r ) + g12(r r ) r + g22(r r ) + g21(r r ) r + eN uu u · uu v · uu u v · uu u · uu v ) * ) * 4 Differential geometry of surfaces in 3-space But (r r ) = 1 (r r ) = 1 g u · uu 2 u · u u 2 11,1 (r r ) = (r r ) 1 (r r ) = g 1 g v · uu u · v u − 2 u · u v 12,1 − 2 11,2 (r r ) = 1 (r r ) = 1 g u · uv 2 u · u v 2 11,2 (r r ) = 1 (r r ) = 1 g v · uv 2 v · v u 2 22,1 (r r ) = (r r ) 1 (r r ) = g 1 g u · vv u · v v − 2 v · v u 12,2 − 2 22,1 (r r ) = 1 (r r ) = 1 g v · vv 2 v · v v 2 22,2 so we have r = 1 g11g + g12g 1 g12g r uu 2 11,1 12,1 − 2 11,2 u + 1 g21g + g22g 1 g22g r + eN ) 2 11,1 12,1 − 2 11,2* v From the definition of the) Christoffel symbols * Γ i = 1 gim g + g g jk 2 jm,k km,j − jk,m we have + , Γ 1 = 1 g11g + g12g 1 g12g 11 2 11,1 12,1 − 2 11,2 Γ 2 = 1 g21g + g22g 1 g22g 11 2 11,1 12,1 − 2 11,2 so by this and similar arguments we arrive finally at the gauss equations4 1 2 r11 = Γ 11r1 + Γ 11r2 + eN 1 2 r12 = Γ 12r1 + Γ 12r2 + f N (4.1) 1 2 r22 = Γ 22r1 + Γ 22r2 + gN The subscripted notation tends to obscure what has here been accomplished; it is perhaps more vividly informative to write 1 2 ruu = Γ 11ru + Γ 11rv + eN 1 2 ruv = Γ 12ru + Γ 12rv + f N (4.2) 1 2 rvv = Γ 22ru + Γ 22rv + gN The Gauss equations are linear, and—because they involve N —extrinsic. In the next section we proceed from the lemma, by a similar argument, to a pair of equations that were introduced in 1861 by J. Weingarten (1836–1910). 4 The occurance of Christoffel symbols in these equations is a bit of an anachronism, since it was mainly during the years 1820–1830 that Gauss (1777–1855) concerned himself with differential geometry, and he had been dead for fourteen years by the time Elwin Christoffel (1829 –1900) introduced the symbols that bear his name. The Weingarten & Mainardi-Codazzi equations 5 The Weingarten equations. These develop N u and N v as linear combinations ofe ru and rv. We expect such formulae to exist, since the unit vector N stands normal to Σ at r, and (by differentiation of N N = 1) N N = N N = 0 so · u· v· N and N lie in the plane tangent to Σ at r, whic· h is spanned· by r· , r . To u v { u v} construct such formulae we return to (3), set a ru, b rv, c N as before but this time look to the cases x N else N .→We then→have → → u v N = g–1 g (r N ) g (r N ) r + g–1 g (r N ) g (r N ) r u 22 u · u − 12 v · u u 11 v · u − 12 u · u v + (N N )N ) * ) · u * N = g–1 g (r N ) g (r N ) r + g–1 g (r N ) g (r N ) r v 22 u · v − 12 v · v u 11 v · v − 12 u · v v + (N N )N ) * ) · v* which by g g E F r N r N e f 11 12 = , u · u u · v = g g F G r ·N r ·N −f −g % 21 22 & % & % v · u v · v & % − − & g–1 = (EG F 2)–1 and N N = N N = 0 give − u· v· fF eG eF fE N u = − ru + − rv EG F 2 EG F 2 (5) gF −fG fF − gE N v = − ru + − rv EG F 2 EG F 2 − − which are the (extrinsic linear) weingarten equations.
Details
-
File Typepdf
-
Upload Time-
-
Content LanguagesEnglish
-
Upload UserAnonymous/Not logged-in
-
File Pages36 Page
-
File Size-