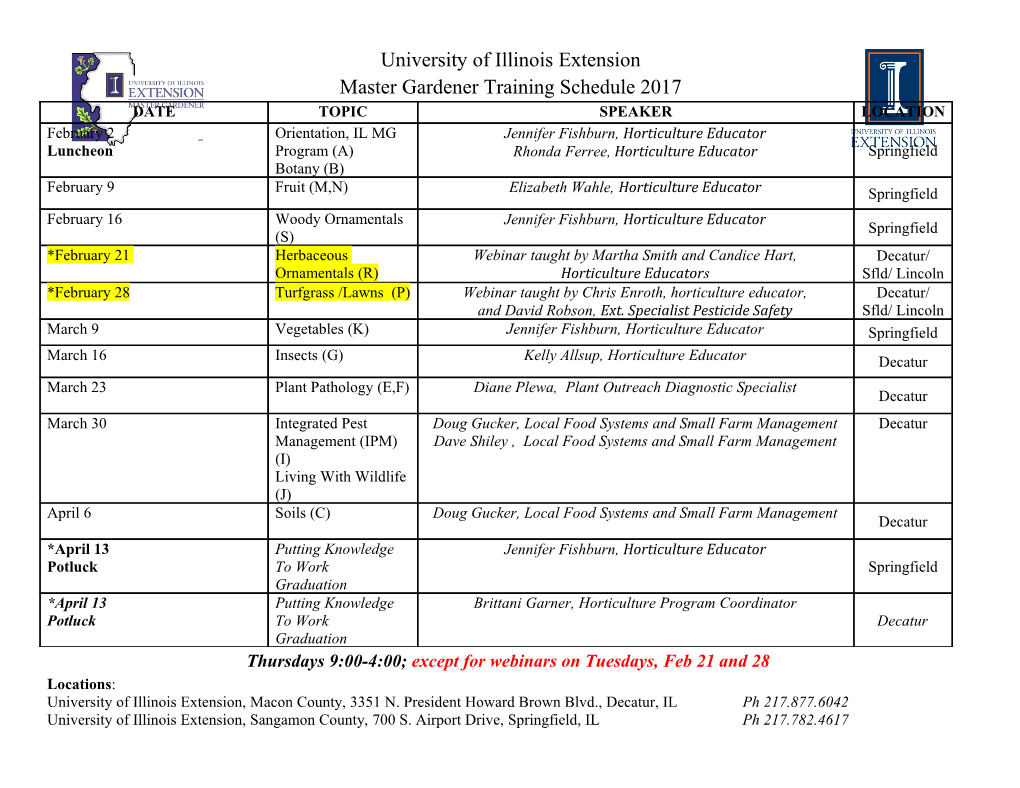
Valorization of Willow Lignin Recovered in a Pilot-scale Biorefinery Based on Hot Water Extraction Aditi Nagardeolekar, Mathew Ovadias, Kuo‐Ting Wang, Christopher Wood and Biljana Bujanovic* SUNY‐ESF, Department of Paper and Bioprocess Engineering, Syracuse, NY – 13210. Introduction: The United States consumed a record 101 quadrillion British thermal units (Btu) worth of energy in the year 2018. Fossil fuels contributed to 80% of this energy. Primary sources of energy under focus in the US have undergone several changes over the course of history. These include wood in the 18th and 19th centuries, later coal and petroleum, followed by renewable sources, such as nuclear energy in the 20th century and more recently, modern renewable sources, such as hydropower, biomass, wind and solar in the 21st century. Modern renewable sources contributed to ~11.4% of the total energy consumption in the year 2018[1]. With energy research becoming increasingly focused on the renewable resources, coal consumption has fallen to the lowest since year 1970[1]. Biomass has been the largest renewable energy source for the last several years, making up around 44% of the total renewable energy and ~5% of the total energy consumed in the US in the year 2018[2]. It is estimated that ~331 million Mg of biomass is used annually in the US for energy production with an additional 750 million to 1 billion Mg of biomass being potentially available, while still meeting food, feed, and fiber demands[3]. The world‐ wide interest in biomass as an energy source has largely been stimulated by the heightened threat of climate change caused by emission of greenhouse gases. Latest agricultural growth has enabled biomass surplus, while the technological growth has enabled higher biomass to energy conversion efficiency[4]. Several types and species of biomass have been suggested as potential energy crops. An ideal energy crop is expected to have following characteristics: high yield of dry biomass per hectare, low cost and energy input to produce, low nutrient requirements, and low amounts of contaminants within the biomass[4]. Short rotation woody plants such as willow (Salix spp.) and herbaceous plants such as perennial grass miscanthus (Miscanthus spp.) fit well into these criteria. Previous experiments in our research group have focused on utilization of miscanthus biomass[5], while current experiments focus on willow. Willow, a short rotation coppice has been increasingly researched as a dedicated energy crop in the US over the past several years, e.g. as a part of the Salix Consortium’s Willow Biomass project, under which willow biomass has been planted in the Northeastern and Midwestern regions of the US[6]. Commercial willow biomass has been planted over 500 hectares in the Northeast region alone[3]. In the NY State, the average yield of Salix clones is 13.6 odt/ha/year, with minimal chemical and energy input (net energy ratio of 55)[6]. In addition to the high yields that can be sustained in 3‐4 years rotation, willow has a favorable chemical composition and energy content (average 8340 Btu/dry pound of three‐ year old willow stems)[7]. It also offers an easy propagation from dormant hardwood cuttings, wide genetic base for selective breeding, and an ability to sprout back after multiple harvests. Therefore, it is suitable to select for specific characteristics and to sustain[8]. In addition to energy provision, willow biomass is expected to provide economical benefits such as creation of new markets and jobs in rural areas. Also, it allows an array of environmental benefits, such as enhanced landscape diversity and wildlife habitat, increased erosion resistance due to its perennial nature and extensive fine root systems, carbon sequestration, significant reduction in greenhouse gas, NOx, SO2 and particulate emissions during energy production and land remediation[3][6][7][8]. Willow, however, is a lignocellulosic biomass (typical composition: extractives 2‐10%; lignin 20‐26%; glucose 35‐42%; xylose 20‐25%; mannose, galactose, arabinose, glucuronic acid 3‐6%; acetic acid 3‐4%)[9] and hence, presents recalcitrance toward the chemical and/or mechanical treatments employed to harness the energy and the chemicals within the biomass. In this report, we outline the results of applying hot‐water extraction (HWE) as the first step toward utilization of willow biomass for the production of a wide range of products, including chemicals and materials/polymers, in biorefineries. Hot‐water extraction (autohydrolysis) is a suggested pretreatment ideal for xylan‐rich angiosperms, viz. hardwoods and grasses[10]. It is an environmentally preferred process, which only uses water at subcritical conditions, thus eliminating the costs associated with use and recovery of harmful chemicals, and which has moderate energy requirements in contrast to supercritical hydrothermal treatments. Its goal is to selectively remove xylan‐based hemicelluloses, by up to 80% of the original xylan content, while leaving cellulose mostly intact[10,11]. Lignin is also partially removed during this process[12]. Applications of cellulose and hemicelluloses have been well explored in literature. The cellulose‐enriched biomass can be utilized in a wide range of cellulose fiber based applications, while the removed xylan‐based carbohydrates can be recovered from the hydrolyzate and further utilized to produce an array of chemicals such as ethanol, furfural, lactic acid and polyhydroxyalkanoates [10,13,14]. Lignin on the other hand has been traditionally underutilized, mainly treated as a waste and burned for energy[15]. However, lignin is the most abundant naturally occurring aromatic polymer, which offers a range of favorable characteristics: a large number of chemically‐reactive sites, film‐forming ability, high carbon content, compatibility with a large number of industrial chemicals, good UV and mechanical stability, moderate thermal stability, good rheological and viscoelastic properties and availability in high volumes at relatively low cost[15]. Considering a critical need to increase use of lignin in biorefineries, this report also outlines the exploratory results of lignin recovered from willow hot‐water hydrolysate for use in synthesis of hydrogels. These hydrogels were synthesized for potential applications in agriculture (for sustained release of bioactive solutions, such as pesticides, fertilizers, and pharmaceuticals) and in wastewater treatment (by selective adsorption of textile dyes and metal ions). Additionally, this lignin has also been evaluated for the production of thermoplastic materials in blends with polylactic acid (PLA), one of the most commercially available biopolymers. Lignin‐PLA blends may be utilized as a packaging material with lignin being an antioxidant and providing UV absorbing functionality[16–18]. Both of these properties would extend the lifetime of the material by preventing free radicals and UV light from degrading PLA[19]. Willow lignin was explored as recovered from the hydrolysate of hot water extraction of willow, and also after esterification, which was performed to improve lignin compatibility with PLA. Lignin esterification replaces the free hydroxyl groups, which are thought to inhibit compatibility with plastics, with a hydrocarbon chain through an ester linkage. Two methods of esterification were considered to improve lignin compatibility with PLA in the production of a thermoplastic blend. These two methods included a short‐chain esterification, acetylation (C2–saturated hydrocarbon chain), and a long‐chain fatty acid esterification with lauric acid (C12–saturated hydrocarbon chain), as described in later sections. The reported studies have a goal to suggest applications for lignin as an underutilized component of willow biomass, in order to promote willow, a short rotation energy crop (SRWC), as a resource for not only energy, but also for novel bio‐based products for additional revenues in a modern biorefinery. Experimental Materials and Methods: 1. Biomass: Willow biomass (Salix spp., Family: Salicaceae; a blend of commercial cultivars with bark) was provided by Dr. T. Volk from SUNY‐ESF Tully Field Station. The biomass was milled into approximately 2 cm long chips and was stored covered in super sacks until it was used for the experiment, at an average moisture content of 20.1%w/w. 2. Pilot Scale HWE of Willow Biomass: HWE was performed in a 65ft3 capacity stainless steel lined digester (Struthers‐Well, Santa Fe Springs, CA, USA; one experiment), at 160oC, for 2 hours, with P‐factor of 691 (Table 1)[20]. The digester was heated with a combination of direct steam injection and indirect heating system. Table 1: Loading and operating conditions for hot‐water extraction of willow biomass Variable Value Biomass chip size ~20mm Biomass loading weight 244.21 kg OD Deionized water/biomass 4.5 Time to ramp up to 160oC 51 minutes The extracted chips were washed with tap water of volume equivalent to that of the hydrolyzate. The resultant biomass was then air dried, and ground to #30 mesh particle size for further characterization. 3. Willow Lignin Recovery from the Hot‐water Hydrolyzate: The hydrolyzate in the storage tank was acidified to pH 2 with 20% sulfuric acid to precipitate the dissolved lignin. The precipitation process was aided by storing the acidified hydrolyzate in a cold room at 8oC for several days. The precipitated lignin was separated from the supernatant by decantation. The lignin recovered from the willow hot‐water hydrolyzate (henceforth
Details
-
File Typepdf
-
Upload Time-
-
Content LanguagesEnglish
-
Upload UserAnonymous/Not logged-in
-
File Pages51 Page
-
File Size-