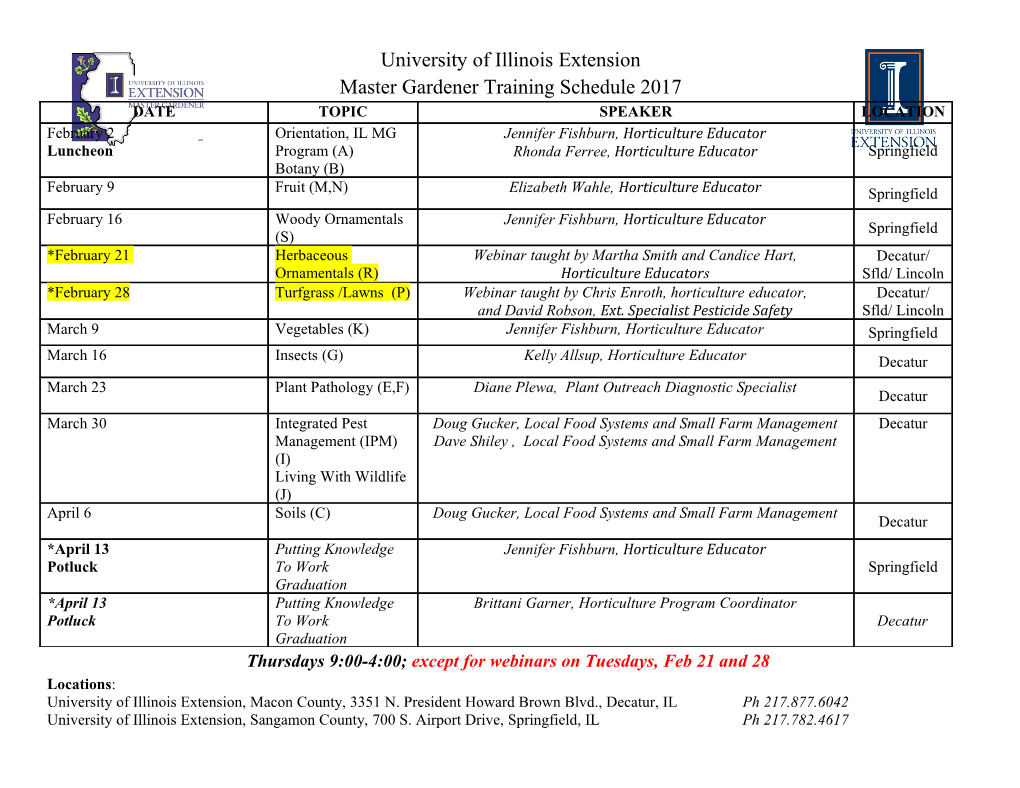
Wright et al. BMC Genomics (2015) 16:791 DOI 10.1186/s12864-015-2020-4 RESEARCH ARTICLE Open Access Development of a SNP-based assay for measuring genetic diversity in the Tasmanian devil insurance population Belinda Wright1, Katrina Morris1, Catherine E. Grueber1,2, Cali E. Willet1, Rebecca Gooley1, Carolyn J. Hogg3, Denis O’Meally1, Rodrigo Hamede4, Menna Jones4, Claire Wade1 and Katherine Belov1* Abstract Background: The Tasmanian devil (Sarcophilus harrisii) has undergone a recent, drastic population decline due to the highly contagious devil facial tumor disease. The tumor is one of only two naturally occurring transmissible cancers and is almost inevitably fatal. In 2006 a disease-free insurance population was established to ensure that the Tasmanian devil is protected from extinction. The insurance program is dependent upon preserving as much wild genetic diversity as possible to maximize the success of subsequent reintroductions to the wild. Accurate genotypic data is vital to the success of the program to ensure that loss of genetic diversity does not occur in captivity. Until recently, microsatellite markers have been used to study devil population genetics, however as genetic diversity is low in the devil and potentially decreasing in the captive population, a more sensitive genotyping assay is required. Methods: Utilising the devil reference genome and whole genome re-sequencing data, we have identified polymorphic regions for use in a custom genotyping assay. These regions were amplified using PCR and sequenced on the Illumina MiSeq platform to refine a set a markers to genotype the Tasmanian devil insurance population. Results: We have developed a set of single nucleotide polymorphic (SNP) markers, assayed by amplicon sequencing, that provide a high-throughput method for monitoring genetic diversity and assessing familial relationships among devils. To date we have used a total of 267 unique SNPs within both putatively neutral and functional loci to genotype 305 individuals in the Tasmanian devil insurance population. We have used these data to assess genetic diversity in the population as well as resolve the parentage of 21 offspring. Conclusions: Our molecular data has been incorporated with studbook management practices to provide more accurate pedigree information and to inform breeding recommendations. The assay will continue to be used to monitor the genetic diversity of the insurance population of Tasmanian devils with the aim of reducing inbreeding and maximizing success of reintroductions to the wild. Keywords: Next-generation sequencing, Single nucleotide polymorphism, Captive breeding, Population management, Pedigree assessment * Correspondence: [email protected] 1University of Sydney, Faculty of Veterinary Science, Rm 303, RMC Gunn Building, Sydney, NSW 2006, Australia Full list of author information is available at the end of the article © 2015 Wright et al. Open Access This article is distributed under the terms of the Creative Commons Attribution 4.0 International License (http://creativecommons.org/licenses/by/4.0/), which permits unrestricted use, distribution, and reproduction in any medium, provided you give appropriate credit to the original author(s) and the source, provide a link to the Creative Commons license, and indicate if changes were made. The Creative Commons Public Domain Dedication waiver (http://creativecommons.org/publicdomain/zero/1.0/) applies to the data made available in this article, unless otherwise stated. Wright et al. BMC Genomics (2015) 16:791 Page 2 of 11 Background Single nucleotide polymorphisms (SNPs) occur through- The Tasmanian devil (Sarcophilus harrisii)hasrecentlysuf- out the genome. SNPs are typically biallelic, and unlike fered a severe population decline due to the recently microsatellites with many alleles at each locus, the number emerged devil facial tumour disease (DFTD) [1]. DFTD is a of loci is a critical consideration when assessing the power rare form of cancer that is transmissible, with tumour cells of a marker set. Between 30 and 80 SNP loci are sufficient acting directly as the infectious agent [2]. DFTD is spread to detect moderate to low population structure, although amongst devils when they bite each other during social inter- more loci may be needed to detect subtle population differ- actions [3] with the biter, rather than the bitten, being more entiation [26]. For parentageandpedigreestudies60–100 likely to become infected [4]. The spread of DFTD has been SNPs are expected to provide sufficient resolution to con- rapid and extensive, covering more than 85 % of the devils’ struct accurate pedigrees, with likelihood-based estimates range since it was first observed in 1996 [5], with localised being more efficient than exclusion-based methods for declines on the east coast of Tasmania of 90 % [1]. Initially it pedigree construction [27]. For any given population, the was suggested that extinction in the wild could occur within number of individuals sampled and number of loci geno- 25–30 years [1], however disease has been present for almost typed must be optimised to achieve appropriate statistical 20 years on the east coast without any documented evidence power to detect a given effect [28]. As SNPs are ubiquitous of localised extinction (Save the Tasmanian Devil Program, in the genome and inexpensive to assay, adding further pers. comm.). The Tasmanian devil also suffers from low SNP loci to a marker set is relatively simple and can genetic diversity at both neutral and functional loci [6–8]. mitigate against the lack of allelic diversity common to The devil is listed as endangered due to the disease [9] and a small or inbred populations. SNPs are known to have a low captive breeding program has been established to prevent genotyping error rate [29] and allele calling is generally extinction of the species. This insurance program has the more consistent than for microsatellites. These features aim of preserving 95 % of founding genetic diversity make SNPs practical for high-throughput genotyping and for a period of 50 years [10]. data sharing across laboratories and organisations [27]. Captive breeding programs have often achieved limited With the ever-decreasing costs of next-generation success because in many cases the species of conservation sequencing (NGS), large-scale sequencing projects in con- concern already shows reduced genetic diversity and/or servation programs are becoming increasingly feasible. small population size. Small population size can result in a Sequencing of PCR amplicons has benefitted greatly from founder effect, and potentially lead to loss of fitness via high-throughput next-generation sequencing technology inbreeding depression [11, 12]. Genetic adaptation to cap- and it is now possible to simultaneously sequence large tivity [13, 14] and further loss of genetic diversity in numbers of samples at multiple loci [30]. In species with a captive populations [15] can occur, leading to decreased reference genome, it is possible to distribute markers fitness upon reintroduction to the wild [16, 17]. To avoid across the genome and target protein coding regions to inbreeding depression and maintain adaptive potential in gain true insights of genome-wide and genic diversity. captivity, breeding interactions are managed to maximize Within the Tasmanian devil insurance population, our re- retention of genetic diversity. Traditionally this has been search group has observed that microsatellite markers have achieved through either pedigree management using stud- often been unable to differentiate between closely related books or more recently using neutral markers such as individuals [31]. We have now taken an amplicon-based microsatellites [15]. There is a move now to integrate approach to target SNPs dispersed across the devil genome. studbook information and molecular data [18], and early We have used these target SNPs to examine genetic diver- studies have highlighted issues of using studbook-based sity within the Tasmanian devil insurance population and management alone, as ancestries are often incomplete, tested whether they provide more accurate discrimination unknown or inaccurate [19–21]. of familial relationships than traditional microsatellite Some have questioned which types of markers are most markers. The Tasmanian devil genome has been published informative to assess and manage diversity of threatened [8, 32] enabling us to target genes of interest in addition to populations [22–24]. Allelic diversity is essential to the regions with putatively neutral variation. Sequencing func- efficacy of multi-allelic markers such as microsatellites, tional regions of the genome enables us to not only assess especially at the level of individual discrimination. Loss of genetic diversity and relatedness but also to make predic- alleles over time in small populations may decrease the tions about the potential functional consequences of allelic utility of such markers in captive breeding programs when loss that may occur as a result of captive breeding. it is necessary to determine relatedness between individ- uals. Another potential disadvantage of microsatellite Results markers is in their inability to make predictions of the SNP discovery and pilot study functional consequences of loss of alleles over time in Re-sequencing data from 7 whole genomes were added captivity [25]. to 3 existing genome sequences (including the reference) Wright et al. BMC Genomics (2015) 16:791 Page 3 of 11 to form the ascertainment
Details
-
File Typepdf
-
Upload Time-
-
Content LanguagesEnglish
-
Upload UserAnonymous/Not logged-in
-
File Pages11 Page
-
File Size-