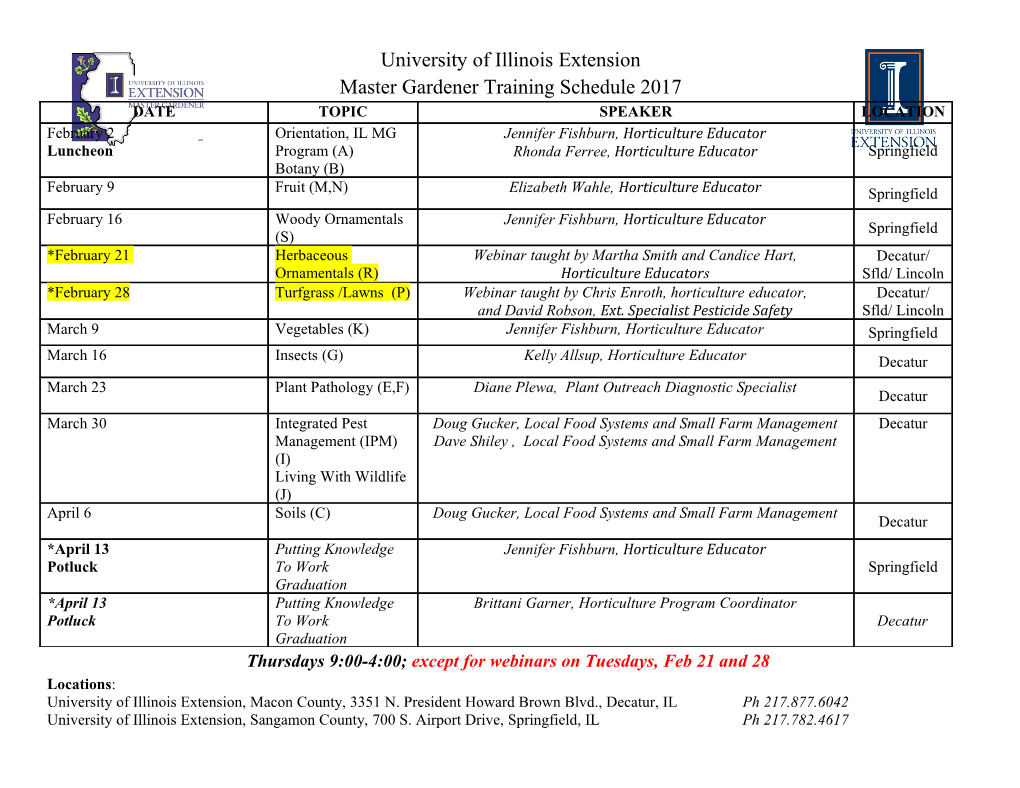
THE SEARCH FOR DARK MATTER: FROM COLLIDERS TO DIRECT DETECTION EXPERIMENTS A DISSERTATION SUBMITTED TO THE DEPARTMENT OF PHYSICS AND THE COMMITTEE ON GRADUATE STUDIES OF STANFORD UNIVERSITY IN PARTIAL FULFILLMENT OF THE REQUIREMENTS FOR THE DEGREE OF DOCTOR OF PHILOSOPHY Mariangela Lisanti July 2010 © 2010 by Mariangela Lisanti. All Rights Reserved. Re-distributed by Stanford University under license with the author. This work is licensed under a Creative Commons Attribution- Noncommercial 3.0 United States License. http://creativecommons.org/licenses/by-nc/3.0/us/ This dissertation is online at: http://purl.stanford.edu/nm932yy4639 ii I certify that I have read this dissertation and that, in my opinion, it is fully adequate in scope and quality as a dissertation for the degree of Doctor of Philosophy. Jay Wacker, Primary Adviser I certify that I have read this dissertation and that, in my opinion, it is fully adequate in scope and quality as a dissertation for the degree of Doctor of Philosophy. Savas Dimopoulos I certify that I have read this dissertation and that, in my opinion, it is fully adequate in scope and quality as a dissertation for the degree of Doctor of Philosophy. Michael Peskin Approved for the Stanford University Committee on Graduate Studies. Patricia J. Gumport, Vice Provost Graduate Education This signature page was generated electronically upon submission of this dissertation in electronic format. An original signed hard copy of the signature page is on file in University Archives. iii iv Acknowledgements My time in graduate school has been a very rewarding experience primarily because of my research group and advisor. I have been very lucky to work with Jay Wacker, whose mentorship and guidance has helped me grow as a research scientist. Our collaboration has introduced me to new and exciting physics and has challenged me to approach problems critically and deeply. Working in Jay's group has always been great fun: many thanks go out to Daniele Alves, Siavosh Behbahani, Anson Hook, Eder Izaguirre, Martin Jankowiak, and Tomas Rube, for all the interesting conversations and laughs. I have had the opportunity to work with many brilliant and wonderful collabo- rators during my time at Stanford: Daniele Alves, Johan Alwall, Roger Blandford, Andy Haas, My Phuong Le, Louis Strigari, Jay Wacker, and Risa Wechsler. I have learned new physics and research tools from each, and feel honored to have had the opportunity to work with them. I would also like to thank my reading committee (Savas Dimopoulos, Michael Peskin and Jay Wacker), as well as my oral committee (Joanne Hewett, Michael Peskin, Jelena Vuckovic, Jay Wacker, and Risa Wechsler) for their time in helping me meet the final requirements to graduate. I am especially grateful to Michael Peskin, who pulled me aside one day after field theory class five years ago and suggested I consider research in particle physics. That simple suggestion gave me the courage to explore a new field of physics with great excitement. During my time at Stanford, I have made wonderful friends with whom I have shared great adventures. To all those who have shared in these experiences with me, especially my friends, family, and Pete: thank you for your continual support and encouragement. v Contents Acknowledgements v 1 Introduction 1 1.1 The Standard Model and Beyond . 3 1.2 Collider searches for dark matter . 7 1.2.1 Jets + ET Channel . 11 6 1.2.2 Discovery through the Higgs Sector . 13 1.3 Direct Detection Experiments . 15 1.3.1 CiDM and Directional Detection . 21 1.4 Summary . 24 2 Collider Searches for Jets+ET 25 6 2.1 Event Generation . 27 2.1.1 Signal . 27 2.1.2 Backgrounds . 29 2.2 Projected Reach of Searches . 30 2.3 Conclusions and Outlook . 33 3 Model-Independent Jets+ET 35 6 3.1 Overview of Models . 38 3.2 Proposed Analysis Strategy . 40 3.3 Event Generation . 45 3.3.1 Signal . 45 3.3.2 Backgrounds . 49 vi 3.4 Gluino Exclusion Limits . 52 3.4.1 No Cascade Decays . 52 3.4.2 Cascade Decays . 54 3.4.3 t-channel squarks . 55 3.4.4 Monophoton Search . 56 3.4.5 Leptons . 58 3.5 Conclusion . 59 4 Dark Matter via the Higgs Sector 61 4.1 Light a0 Modifications to Higgs Phenomenology . 63 4.2 h0 a0a0 at Hadron Colliders . 71 ! 4.2.1 Signal . 72 4.2.2 Backgrounds . 74 4.2.3 Expected Sensitivity . 81 4.3 Conclusion . 81 5 Prospects for Inelastic Dark Matter 83 5.1 Inelastic Dark Matter at CRESST . 86 5.2 XENON100 Prospects . 91 5.3 Conclusions . 92 6 Six Higgs Doublet Model 93 6.1 The Model . 95 6.2 RG Influence on Low Energy Spectrum . 98 6.2.1 Gauge Unification . 98 6.2.2 Quartic Couplings . 99 6.3 Dark Matter . 103 6.3.1 Relic abundance . 103 6.3.2 Bounds from electroweak precision tests . 104 6.4 Experimental Signatures . 106 6.4.1 Direct detection . 106 6.4.2 Indirect detection . 108 vii 6.4.3 Collider Signatures . 110 6.5 Conclusions . 114 7 Parity Violation in CiDM Models 116 7.1 Models of CiDM . 117 7.1.1 Axially Charged Quarks . 119 7.1.2 Vectorially Charged Quarks . 121 7.1.3 Parity Violation . 122 7.2 Direct Detection Phenomenology . 123 7.3 Searches for the Dark Photon . 129 7.3.1 Limits from Direct Production . 131 7.4 Discussion . 134 8 Directional Detection 137 8.1 Direct Detection Phenomenology . 139 8.2 Directional Detection . 146 8.3 Discussion . 150 Bibliography 152 viii List of Tables 2.1 Selection criteria for jets +ET searches . 31 6 3.1 Differential cross section for background and signal . 44 4.1 Relative and cumulative signal efficiencies . 73 4.2 Continuum backgrounds for low invariant mass muon pairs . 75 7.1 Benchmark CiDM Models . 128 ix List of Figures 1.1 Rotation Curve . 2 1.2 The Standard Model . 4 1.3 Schematic of dark matter freeze-out . 6 1.4 Radiative corrections to the Higgs mass . 8 1.5 MSSM particle content. 10 1.6 Gluino decays . 12 1.7 Direct detection experiments for spin-independent dark matter. 15 1.8 Recoil energy spectra . 19 1.9 DAMA's modulated amplitude . 20 2.1 Boosted gluinos . 27 2.2 The 95% gluino-bino exclusion curve for D0 at 4 fb−1 . 32 6 3.1 Comparison of D0 cuts and optimized cuts for a sample dijet signal . 41 6 3.2 Differential 0 1 jet rate for a matched sample of light gluino production 48 ! 3.3 The importance of matching . 51 3.4 Differential cross section for theoretical model spectrum . 52 3.5 The 95% exclusion region for D0 at 4 fb−1 . 53 6 3.6 Exclusion plot for cascading gluinos . 54 3.7 Gluino production cross section as a function of squark mass . 56 4.1 Branching fraction of Higgs to pseudoscalars . 67 4.2 Values of S = sin 2β (GeV) excluded by LEP2 . 69 h i 4.3 Parameter space excluded by CLEO . 70 x 4.4 Schematic of Higgs decay chain . 74 4.5 Muon invariant mass before and after cuts . 76 4.6 Total invariant mass . 77 4.7 Expected sensitivity to Higgs production cross section . 79 5.1 CRESST recoil spectra . 87 5.2 Average counts at CRESST . 88 5.3 Average counts at XENON100 . 90 6.1 Allowed range for of quartic couplings . 101 6.2 Allowed mass of the LSP in the six Higgs double model . 105 6.3 Direct detection possibilities for six Higgs doublet model . 107 6.4 Flux from dark matter annihilation in six Higgs doublet model . 109 6.5 Dark matter mass splitting . 111 6.6 Width of the SM Higgs decay . 112 6.7 LHC production cross section . 114 7.1 Allowed regions in mπ Λd parameter space . 125 d − 7.2 Allowed regions in mπ feff parameter space . 126 d − 7.3 Limits on kinetic mixing . 129 8.1 Modulation amplitude for FFeDM and iDM . 139 8.2 Allowed m δm parameter space . 144 − 8.3 Estimated recoil spectrum at LUX . 146 8.4 Comparison of recoil angle spectra for FFeDM and iDM . 148 8.5 Predicted rates at directional detection experiments . 149 xi xii Chapter 1 Introduction The presence of dark matter in the universe is one of the greatest mysteries of par- ticle physics. While dark matter is believed to play an important role in structure formation, very little is known about its properties. Because the dark matter emits no detectable radiation, its presence has been deduced indirectly through gravita- tional effects. Its existence was first inferred by the astronomer Fritz Zwicky in the 1930s. At the time, Zwicky noted that the predicted mass of the Coma Cluster from luminosity measurements fell markedly short of dynamical estimates of the cluster's mass using the virial theorem [1]. In his paper \On the Masses of Nebulae and of Clusters of Nebulae," Zwicky wrote that \This discrepancy is so great that a further analysis of the problem is in order," however it was not until several decades later that dark matter reemerged as a pressing scientific issue with the.
Details
-
File Typepdf
-
Upload Time-
-
Content LanguagesEnglish
-
Upload UserAnonymous/Not logged-in
-
File Pages179 Page
-
File Size-