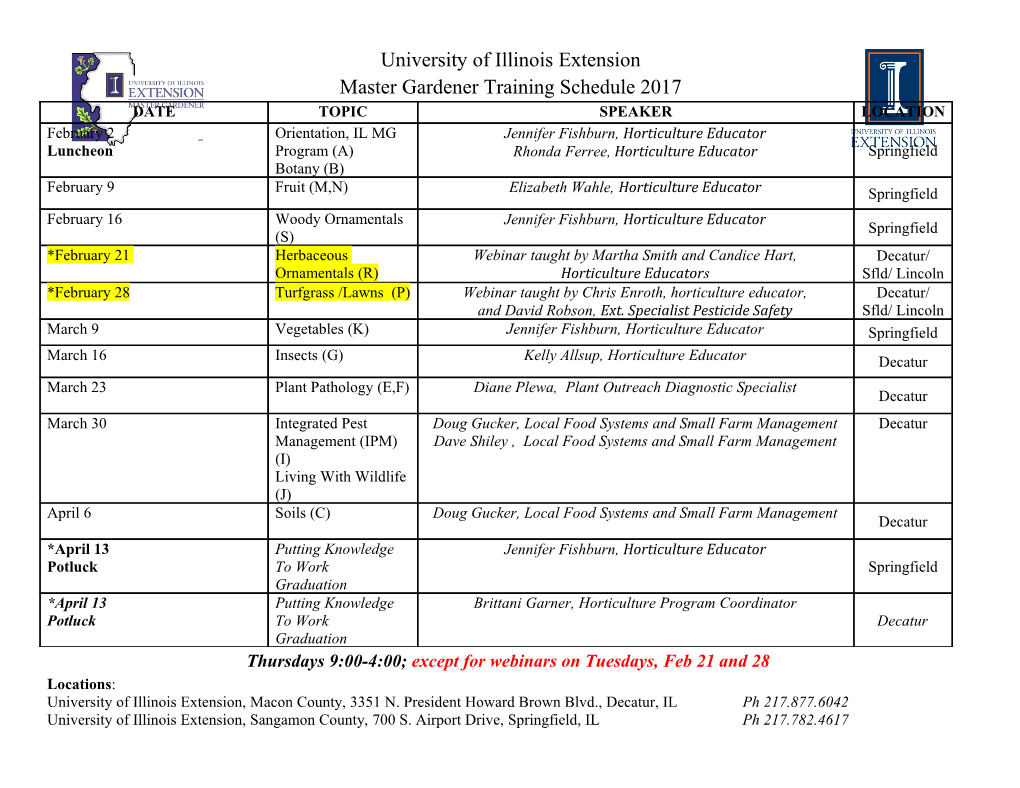
PUBLICATIONS Geophysical Research Letters RESEARCH LETTER Gyre-scale deep convection in the subpolar North 10.1002/2016GL071895 Atlantic Ocean during winter 2014–2015 Key Points: A. Piron1 , V. Thierry1 , H. Mercier2 , and G. Caniaux3 • Exceptional heat loss caused exceptional convection at subpolar 1Ifremer, Laboratoire d’Océanographie Physique et Spatiale, UMR 6523 CNRS-IFREMER-IRD-UBO, Plouzané, France, 2CNRS, – gyre scale during winter 2014 2015 ’ 3 • Exceptionally deep mixed layers Laboratoire d Océanographie Physique et Spatiale, UMR 6523 CNRS-IFREMER-IRD-UBO, Plouzané, France, Centre National directly observed south of Cape de Recherches Météorologiques, UMR 3589 Météo-France-CNRS, Toulouse, France Farewell (1700 m) and in the Irminger Sea (1400 m) • This exceptional convection was Abstract Using Argo floats, we show that a major deep convective activity occurred simultaneously in the favored by and enhanced the cold Labrador Sea (LAB), south of Cape Farewell (SCF), and the Irminger Sea (IRM) during winter 2014–2015. anomaly that developed recently in the North Atlantic Ocean Convection was driven by exceptional heat loss to the atmosphere (up to 50% higher than the climatological mean). This is the first observation of deep convection over such a widespread area. Mixed layer depths Supporting Information: exceptionally reached 1700 m in SCF and 1400 m in IRM. The deep thermocline density gradient limited the • Supporting Information S1 mixed layer deepening in the Labrador Sea to 1800 m. Potential densities of deep waters were similar in the À three basins (27.73–27.74 kg m 3) but warmer by 0.3°C and saltier by 0.04 in IRM than in LAB and SCF, Correspondence to: meaning that each basin formed locally its own deep water. The cold anomaly that developed recently in the V. Thierry, [email protected] North Atlantic Ocean favored and was enhanced by this exceptional convection. Citation: 1. Introduction Piron, A., V. Thierry, H. Mercier, and G. Caniaux (2017), Gyre-scale deep con- Deep convection is a key oceanic process that ventilates the lower limb of the Meridional Overturning vection in the subpolar North Atlantic Circulation (MOC), thus contributing to the storage of heat, anthropogenic carbon and oxygen in the deep – Ocean during winter 2014 2015, ocean [Pérez et al., 2013]. Monitoring and understanding variability in volumes and properties of the water Geophys. Res. Lett., 44, 1439–1447, doi:10.1002/2016GL071895. masses produced by deep convection is required to better model and predict future changes in deep con- vection in a warming world. It would also allow a better assessment of the link between deep convection Received 10 NOV 2016 and the MOC intensity measured at mid-latitudes [Cunningham et al., 2007] and subpolar latitudes [Mercier Accepted 27 JAN 2017 et al., 2015]. Accepted article online 29 JAN 2017 Published online 13 FEB 2017 The Labrador Sea Water (LSW) is formed by deep convection in the western North Atlantic Ocean subpolar gyre. In the last decades several sites of LSW production were identified, each site showing large interannual to decadal variability in convection strength. In the Labrador Sea, the deepest winter mixed layers were observed between the late 1980s and the mid-1990s [Yashayaev, 2007] due to the recurrence of severe win- ters during this high North Atlantic Oscillation (NAO) index period [Hurrell, 1995]. They reached 2300–2400 m in 1993–1995 [Kieke and Yashayaev, 2015]. Since the mid-1990s, the convection was shallower due to milder winters and the maximum mixed layer depths (MLDs) ranged from 500 to 1500 m, except in 2008 and 2014 when they reached 1850 and 1700 m, respectively [Våge et al., 2009; Yashayaev and Loder, 2009; Kieke and Yashayaev, 2015]. Based on hydrographic data collected over the period 1900–2000, Pickart et al. [2003a] showed that the core of the deep convection area is localized west of 52°W and south of 59°N. The deep con- vection area extended to 48°W and 60°N during the deep convective years of the early 1990s [Pickart et al., 2003a] and retreated westward in the early 2000s [Våge et al., 2009]. In the last decade, the Irminger Sea was also recognized as a deep convection site forming LSW under favor- able conditions such as positive NAO index, preconditioning of the water column, and strong heat loss to the atmosphere due to high wind speed events occurring east of Cape Farewell (the so-called Greenland Tip Jets) [Bacon et al., 2003; Pickart et al., 2003b; Våge et al., 2009; de Jong et al., 2012; Piron et al., 2016]. The end of winter MLDs in the Irminger Sea reached 1000 m in 2008 and 2012 [de Jong et al., 2012; Piron et al., 2016] and 1400 m in 2015 [de Jong and de Steur, 2016; Fröb et al., 2016], which are the deepest mixed layers observed in that region. Based on a one-dimensional (1-D) mixed layer model, Våge et al. [2008] and Pickart et al. [2003a] suggested that convection might have reached 1500–2000 m in 1994 and 1995. South of Cape Farewell, Bacon et al. [2003] and Piron et al. [2016] observed MLDs of 900–1000 m in 1997 and ©2017. American Geophysical Union. 2012, respectively. While this region was considered in some studies as part of the Irminger Sea [Piron et al., All Rights Reserved. 2016; Bacon et al., 2003], it could be a third distinct deep convection site. Indeed, using the minimum PIRON ET AL. NORTH ATLANTIC 2014–2015 DEEP CONVECTION 1439 Geophysical Research Letters 10.1002/2016GL071895 Figure 1. (a) Positions of Argo profiles available over January–April 2015. Black dots: MLD < 700 m; colored dots: MLD > 700 m. Superimposed are the 1000 m isobath (thin black line) and the Absolute Dynamic Topography (cm) aver- aged over January–April 2015 (gray contours). (b) Positions of the late winter (March–April) MLD > 1000 m in the LAB (blue dots), SCF (green dots), and IRM (red dots) boxes. Black dots: the deepest mixed layer in each box (1790, 1700, and 1400 m, respectively). Yellow dots: profiles with MLD > 1000 m outside the boxes. Black circles: profiles with oxygen data shown in Figure 3. Black contours: winter (“”DJFM) anomalies (in %) of air-sea heat fluxes relative to the 1979–2015 climatological value. Positive numbers indicate stronger than average air-sea heat loss to the atmosphere. potential vorticity at 1000 m as a footprint of the deep convective activity, Pickart et al. [2003a] showed that during the 1989–1997 period there was a separate area of minimum potential vorticity south of Cape Farewell. In this latter area, a near-surface stratified layer capping a homogenous layer down to 1800 m in 1991 and 1600 m in 2008 was observed by Pickart et al. [2003a] and Våge et al. [2009], respectively. Those results suggest that MLDs could extend deeper than 1000 m south of Cape Farewell. Våge et al. [2009] described the deep convection at the subpolar gyre scale for the winter 2007–2008 (winter (N À 1)–N will be referred to as WinterN) owing to Argo data. The sampling in the Irminger Sea and south of Cape Farewell was, however, insufficient to allow comparison of the deep convection properties (depth, spatial extent, and thermohaline properties) in the different convection sites and to relate them to the PIRON ET AL. NORTH ATLANTIC 2014–2015 DEEP CONVECTION 1440 Geophysical Research Letters 10.1002/2016GL071895 Figure 2. (a–c) Late winter (March–April) profiles with MLD > 1000 m in the LAB (Figure 2a), SCF (Figure 2b), and IRM À (Figure 2c) boxes. Thick lines: potential density (kg m 3). Thin dashed lines: oxygen saturation (%). Black line: profiles with the deepest mixed layers. Yellow line: profile located outside the boxes. MLD is indicated with dots on the density profiles. (d) Properties of the late winter profiles with MLD > 1000 m in LAB (blue dots), SCF (green dots), and IRM (red dots). The black circles identify properties of the deepest mixed layers. The yellow dots identify profiles located outside the boxes. atmospheric forcing and oceanic preconditioning. This is now possible for Winter15 because of an adequate Argo sampling of the subpolar gyre. The number of profiles available in the three convection sites during winter (DJFM) was 2 to 3 times larger in Winter15 than in Winter08 (183 profiles against 80). Winter15 was characterized by deep mixed layers [Fröb et al., 2016] and a NAO index close to 2 corresponding to the highest value observed since the early 1990s suggesting a strong atmospheric forcing. This motivated the investigation of deep convection in the subpolar gyre for Winter15. 2. Data and Methods Argo data were used to describe the mixed layers for Winter15. We selected Argo temperature, salinity, and oxygen profiles available in the subpolar gyre of the North Atlantic Ocean (52–66°N; 30°W–66°W) between 1 September 2014 and 30 April 2015 [Argo, 2015]. Oxygen data were corrected as suggested by Takeshita et al. [2013] by using as a reference either the oxygen profile collected at float deployment if available or the World Ocean Atlas 2009 [Garcia et al., 2010]. As in Piron et al. [2016], MLDs were calculated for each profile by com- paring the split-and-merge [Thomson and Fine, 2003] and threshold [de Boyer Montégut et al., 2004] methods and a visual control. Mixed layers isolated from the surface [Pickart et al., 2002] are not considered here to guarantee that the estimated MLDs correspond to mixed layers formed locally. We used the surface wind stress, air-sea heat flux, and sea surface temperature (SST) provided by the ERA- Interim reanalysis [Dee et al., 2011]. We used the Absolute Dynamic Topography (ADT) data provided by AVISO, and Sea Ice data from the National Snow and Ice Data Center (NSIC) [Fetterer et al., 2016].
Details
-
File Typepdf
-
Upload Time-
-
Content LanguagesEnglish
-
Upload UserAnonymous/Not logged-in
-
File Pages9 Page
-
File Size-