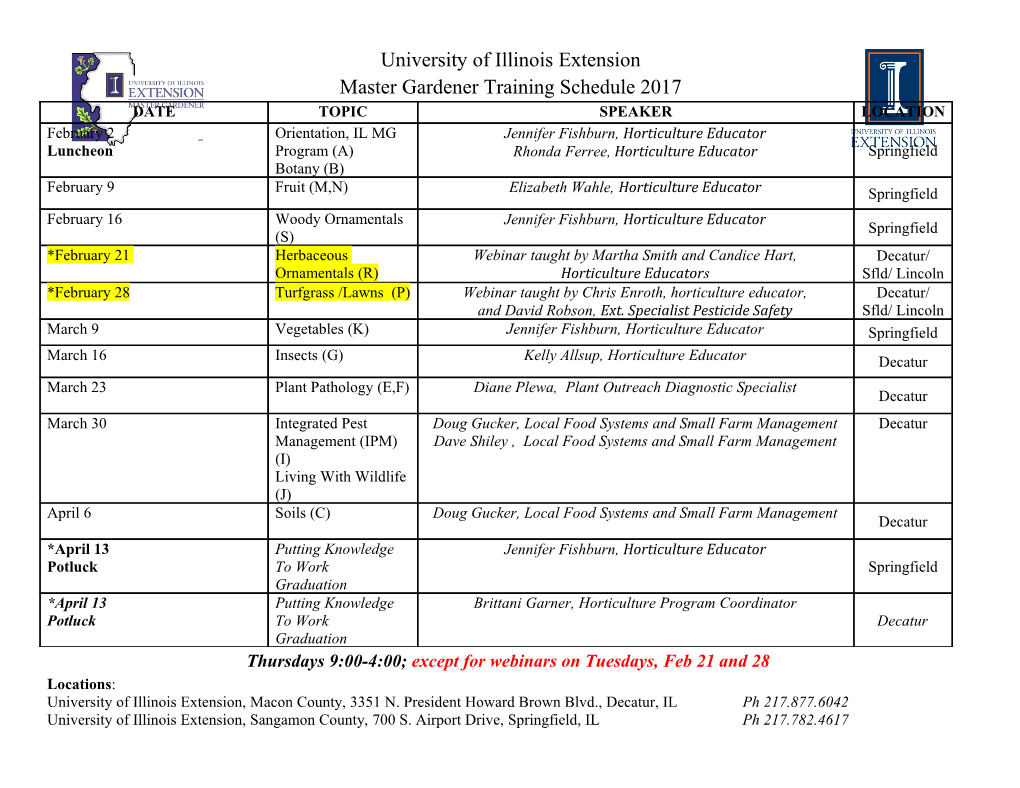
Aerodynamics of Track Cycling By Lindsey Underwood A thesis presented for the Degree of Doctor of Philosophy at The University of Canterbury Department of Mechanical Engineering Christchurch, New Zealand June 2012 i Abstract The aim of this thesis was to identify ways in which the velocity of a track cyclist could be increased, primarily through the reduction of aerodynamic drag, and to determine which factors had the most significant impact on athlete performance. An appropriate test method was set up in the wind tunnel at the University of Canterbury to measure the aerodynamic drag of different cycling positions and equipment, including helmets, skinsuits, frames and wheels, in order to measure the impact of specific changes on athlete performance. Rather than comparing standard cycling positions, such as the Obree, dropped, upright and aero positions, a number of individual and multiple changes were made to the height, width and length of the handlebars, seat height, head and helmet position, and hand position to provide a rank of importance in terms of which changes had the greatest impact (gain or loss) on the aerodynamic drag. A mathematical model of the Individual Pursuit (IP) event was also created to calculate the velocity profile and finishing time for athletes competing under different race conditions. The model was created in Microsoft Excel and used first principles to analyse the forces acting on a cyclist, which lead to the development of equations for power supply and demand. The model included the effects of leaning in the bends, and used SRM power data to determine the actual position of the rider on the track. The mathematical model was validated using SRM data for eleven, elite track cyclists, and was found to be accurate to 0.31s (0.16%). An analysis of changes made to the bike, athlete, and environmental conditions using the mathematical model showed that the drag area and air density had the greatest impact on the finishing time. As the air density is fixed for all athletes competing in the same race, these results suggest that athletes should focus on minimising their drag area in order to maximise performance. The model was then used to predict the finishing times for different pacing strategies by generating different power profiles for a given athlete with a fixed stock of energy (the work done remained the same for all generated power profiles) in order to identify the optimal pacing strategy for the IP. A comparison of the predicted finishing times for the different pacing strategies showed that an all-out and even or all-out and variable strategy with an initial acceleration phase of 12s resulted in the fastest finishing time for a male, IP athlete with a fixed stock of energy whose efficiency is not a function of power output or speed. The length of time spent in the initial acceleration phase was found to have a significant impact on the results, although all strategies simulated with an initial acceleration phase resulted in a faster finishing time than all other strategies simulated. Results from the wind tunnel tests also showed that, in general, changes made to the position of the cyclist had the greatest impact on the aerodynamic drag compared to changes made to the equipment. Multiple changes in position had a greater impact on drag than individual changes in position, but the changes were not additive; the total gain or loss in drag for multiple changes in position was not the sum of individual gains or losses in drag. Actual gains and losses also varied significantly between athletes, primarily due to differences in body size and shape, riding experience, and reference position from which changes were made from. However, certain changes in position or equipment had a significant impact (>±1%) on the drag of all athletes tested compared to the reference position. For example, by wearing a shoe cover all athletes showed a reduction in drag of >1% regardless of shoe, cleat or pedal worn. Changes in position that resulted in a reduction of the frontal area, such as lowering the handlebars and head, were the most successful at reducing the aerodynamic drag, and a change in skinsuit was found to have the greatest impact on drag out of all equipment changes, primarily due to the choice of material and seam placement. The mathematical model was used to quantify the impact of changes in position and equipment made in the wind tunnel on the overall finishing time for a given athlete competing in an IP event. Time savings of up to 8 seconds were seen for ii multiple changes in position, and up to 5 seconds for changes to the equipment. Overall this thesis highlights the significance of aerodynamics on athlete performance in track cycling, sug- gesting that it is worthwhile spending time and money on research and technology to find new ways to reduce the aerodynamic drag and maximise the speed of cyclists. Although this thesis primarily concentrates on the Individual Pursuit event in track cycling, the same principles can be applied to other cycling disciplines, as well as to other sports. iii Acknowledgements I would like to thank Dr Mark Jermy for giving me the opportunity to carry out this research and providing me with support and encouragement throughout my time at the University of Canterbury. My thanks also extend to the technicians Graeme Harris and Eric Cox for assisting me with all the testing and procedures carried out in the wind tunnel, and also to my co-supervisor Stephanie Gutschmidt for her general support. Thanh, Laura and Lisa, I could not have got through the past few years without having such great office mates. Those morning chats are invaluable, and you have provided great friendship and support that I hope will continue when we go our separate ways. Thanks to BikeNZ, SPARC, the REC centre, and all the athletes and coaches who provided data for my thesis, gave me the opportunity to get involved in the High Performance Programme, and helped me attend conferences and competitions to develop my knowledge in sports engineering and track cycling. I must also thank Stuart McIntyre for his outstanding knowledge on apparel design, and for his enthusiam throughout the skinsuit project - you have been a pleasure to work with, and I hope that we can work together again in the future. I would like to show my appreciation to all the international students who have worked with me in the wind tunnel and on projects related to my thesis. Particular thanks must go to Jana, Julien, and Amaury for their hard work and also the entertainment they provided. I am grateful to my flatmates, my friends, and of course my boyfriend, who have all made my time in New Zealand so enjoyable. You have provided me with a home away from home, and a friendship and companionship that I am sure will last a lifetime. Finally my thanks must go to my parents and my brother. Without your continuous love and support, even when I am the other side of the world, I would not be where I am today. iv List of Publications 1. L. Underwood and M.C. Jermy, Mathematical model of track cycling: the individual pursuit, The Engineering of Sport 8, 2 (2): 3217–3222, 2010. 2. L. Underwood and M.C. Jermy, Optimal hand position for individual pursuit athletes, The Engineering of Sport 8, 2 (2): 2425–2429, 2010. 3. L. Underwood and M.C. Jermy, Fabric testing for cycling skinsuits, 5th Asia-Pacific Congress on Sports Technology (APCST), 13: 350-356, 2011. 4. L. Underwood, J. Schumacher, J. Burette-Pommay and M. Jermy, Aerodynamic drag and biomechanical power of a track cyclist as a function of shoulder and torso angles, Sports Engineering, 14 (2-4): 147-154, 2011. 5. L. Underwood and M.C. Jermy, Determining the optimal pacing strategy for the track cycling individual pursuit event with a fixed energy mathematical model, Submitted to Sports Engineering - in review, May 2012. 6. L. Underwood and M.C. Jermy, Optimal handlebar position for individual pursuit athletes, Submitted to Sports Engineering - in review, May 2012. Contents 1 Introduction 1 1.1 Motivation for research . 1 1.2 Aims and Objectives . 1 1.3 Thesis Structure . 2 2 Background 3 2.1 Factors Affecting Power Supply . 7 2.2 Factors Affecting Power Demand . 11 3 Literature Review 13 3.1 Literature Review: Mathematical Model . 13 3.1.1 Types of Models . 14 3.1.1.1 Supply, Demand, and Supply-Demand Models . 14 3.1.1.2 Goodness of Fit and First Principle Models . 15 3.1.2 Existing Models for Cycling . 15 3.1.2.1 Aerodynamic Drag . 16 3.1.2.2 Rolling Resistance . 21 3.1.2.3 Grade Angle / Potential Energy . 23 3.1.2.4 Kinetic Energy . 23 3.1.2.5 Assumptions existing models use . 24 3.1.2.6 Accuracy of existing models . 27 3.1.3 Team Pursuit Models . 27 3.1.4 Pacing Strategy . 29 3.2 Literature Review: Aerodynamics . 34 3.2.1 Background . 34 v CONTENTS vi 3.2.1.1 Pressure Drag, Skin Friction Drag and Base Drag . 34 3.2.1.2 Separation . 37 3.2.1.3 Boundary Layer Theory . 39 3.2.1.4 Tripping the Boundary Layer and the Significance of Surface Roughness . 41 3.2.2 Factors Affecting Aerodynamic Drag of Cycling . 48 3.2.2.1 Athlete Position . 48 3.2.2.2 Drafting . 55 3.2.2.3 Helmets . 56 3.2.2.4 Clothing . 58 3.2.2.5 Pedals and Footwear . 67 3.2.2.6 Bike Frame .
Details
-
File Typepdf
-
Upload Time-
-
Content LanguagesEnglish
-
Upload UserAnonymous/Not logged-in
-
File Pages298 Page
-
File Size-