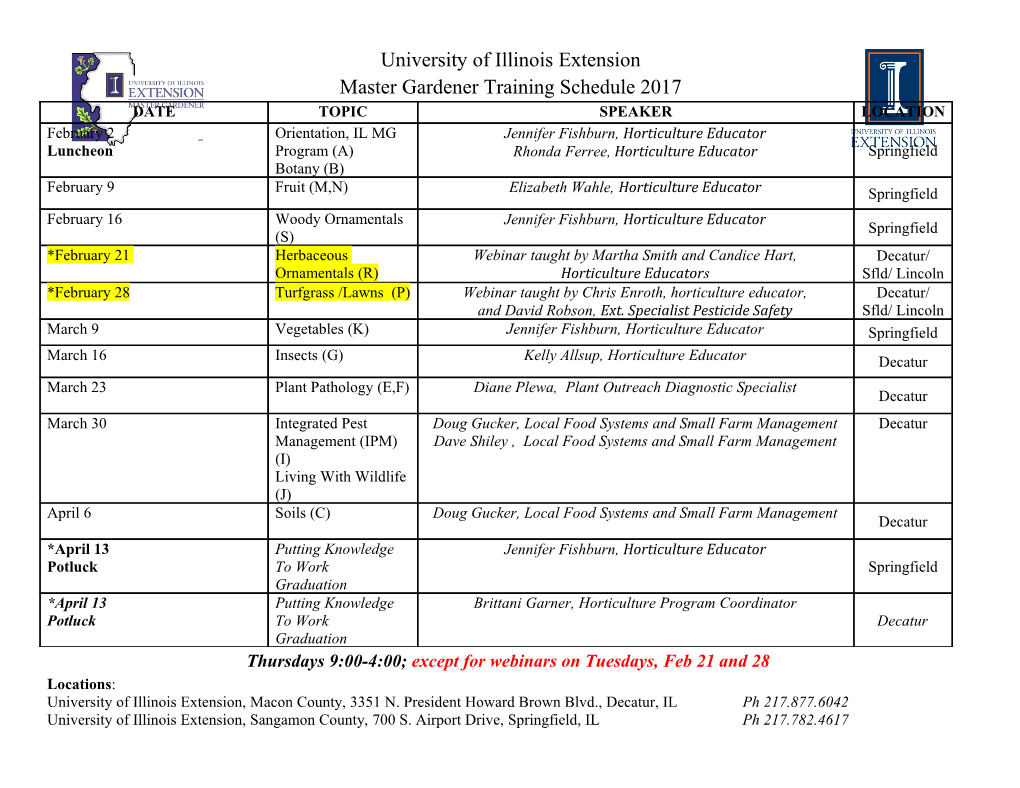
MNRAS 000,1{5 (2019) Preprint 11 March 2019 Compiled using MNRAS LATEX style file v3.0 Planetary Magnetism as a Parameter in Exoplanet Habitability Sarah R.N. McIntyre,1? Charles H. Lineweaver1;2 and Michael J. Ireland1 1Research School of Astronomy and Astrophysics, Australian National University, Canberra, ACT 2611, Australia 2Research School of Earth Sciences, Australian National University, Canberra, ACT 2611, Australia Accepted XXX. Received YYY; in original form ZZZ ABSTRACT Evidence from the solar system suggests that, unlike Venus and Mars, the presence of a strong magnetic dipole moment on Earth has helped maintain liquid water on its sur- face. Therefore, planetary magnetism could have a significant effect on the long-term maintenance of atmosphere and liquid water on rocky exoplanets. We use Olson & Christensen's (2006) model to estimate magnetic dipole moments of rocky exoplanets with radii Rp ≤ 1.23 R⊕. Even when modelling maximum magnetic dipole moments, only Kepler-186 f has a magnetic dipole moment larger than the Earth's, while ap- proximately half of rocky exoplanets detected in the circumstellar habitable zone have a negligible magnetic dipole moment. This suggests that planetary magnetism is an important factor when prioritizing observations of potentially habitable planets. Key words: planetary magnetism { planetary habitability { terrestrial planets 1 INTRODUCTION have lost most of it. Venus' atmosphere has a D/H abun- dance ratio ∼100 D/H of bulk Earth (Lammer et al. 2008). Within the next decade, upcoming observations with near- Without the protection of a magnetic field, water vapour future telescopes will detect an ever-increasing number of photodissociated in the upper atmosphere due to enhanced exo-Earths. In order to make the most of the limited obser- early solar EUV irradiation (Kasting & Pollack 1983). This vational resources available, target selection has focused on led to the escape of hydrogen into space and resulted in rapid `habitable worlds' defined as rocky bodies (with enough sur- surface liquid water loss (Lammer et al. 2011). In 2015 the face gravity to sustain an atmosphere) orbiting their host Maven mission confirmed that water was abundant and ac- stars at a distance where stellar radiation is suitable for tive on Mars for the first few hundred million years of the the presence of surface liquid water (Kaltenegger 2017). solar system (Jakosky et al. 2015). However, at some point However, numerous planetary and astronomical factors in- less than a billion years after Mars formed, its global mag- fluence an exoplanet's ability to maintain liquid water. It netic field went extinct, removing a source of protection from is increasingly important to expand our considerations to the solar winds (Stevenson 2001; Golombek & Phillips 2010). multiple parameters including magnetic field, albedo, stellar The loss of water raised Mars' atmospheric D/H ratio to its type, planet chemical composition, orbital eccentricity, in- current value ∼5 D/H of Earth (Hallis 2017). clination, tidal locking, impact events, and plate tectonics. arXiv:1903.03123v1 [astro-ph.EP] 7 Mar 2019 This will enable us to prioritize planets most likely to main- Almost all of the currently detected rocky exoplanets tain liquid water in order to best utilize telescope time when are on close, highly-irradiated orbits, bombarded by large biosignature observations become a possibility. amounts of ionizing EUV and X-ray radiation (Owen & Most assessments of habitability stem from observations Jackson 2012). With weak or negligible magnetic protec- of our own solar system. The presence of a magnetic dipole tion, the upper atmosphere of an exoplanet will be more moment on Earth protects the surface and liquid water from exposed to stellar winds and coronal mass ejections, result- solar winds and flares (Elkins-Tanton 2013). Venus, Earth ing in atmospheric mass-loss due to non-thermal processes and Mars likely began with similar amounts of water (Grin- such as ion pickup, photo-chemical energizing mechanisms, spoon 1993; Way et al. 2016). This is corroborated by their and sputtering (Vidotto 2013). Furthermore, even in the ab- deuterium to hydrogen ratios (D/H) which suggest that both sence of stellar wind effects, the closed magnetic field lines Mars and Venus had more water early in their histories and above an exosphere inhibit the loss of charged particles to interplanetary space (Lammer et al. 2002). Since planetary magnetism reduces non-thermal atmospheric erosion, it af- ? E-mail: [email protected] fects the evolution of a planet's environment and its po- © 2019 RAS 2 S. R. N. McIntyre et al. tential habitability (Gudel¨ et al. 2014). Here we focus on model the best-case scenario, we have set ROl = 0:12 to gen- modelling the strengths of the magnetic dipole moments of erate an optimal convective buoyancy flux, allowing us to rocky exoplanets to assess their ability to protect their at- estimate the maximum magnetic dipole moment (Mmax). mospheres from hydrogen loss (∼ water loss). 2.2 Rotation rate calculations 2 METHOD Our estimates of rotation rate Ω depend on whether an exo- planet is tidally locked or non-tidally locked. For the subset 2.1 Magnetic moment model description of tidally locked exoplanets we assume that the rotation pe- In our calculations we use the Olson & Christensen(2006) riod equals the orbital period. Grießmeier et al.(2009) was magnetic moment scaling law: used to calculate the time taken to tidally lock an exoplanet: 3 ! 2 6 1/2 4 0 Rp Mp d 3 ρ0 1/3 τsync ≈ αQp Ωi − Ωf (4) M = 4πr0 γ ¹FDº (1) 9 GMp M∗ Rp µ0 where d is the planet's semi-major axis and the structure where M is the magnetic moment (in A m2), r is the 0 parameter α is set at Earth's value of α = 1/3. We adopt planet's core radius, a fitting coefficient γ = 0:15 ± 0:05 is tidal dissipation factor of 0 corresponding to \small inferred from numerical simulations (Olson & Christensen Qp = 500 super-Earths" and \ocean planets" (Grießmeier et al. 2009). 2006), ρ is the bulk density of the outer liquid core (we use 0 The initial and final rotation rates Ω and Ω of a rocky the Earth's density model: ρ = 11000±1100 kg m−3 (Litasov i f 0 exoplanet are not well-known quantities (Correia & Laskar & Shatskiy 2016)), the magnetic permeability of the vacuum 2003). Following our strategy to model the maximum dipole µ = 4π × 10−7H/m, F is the average convective buoyancy 0 magnetic moment, we (i) use a high initial rotation rate flux, and D is the thickness of the outer liquid core. analogous to early Earth's rotation period of 4 hr prior to One of the factors needed for equation (1) is planetary the moon-forming impact, corresponding to Ω : Ω core radius, r . Zeng et al.(2016) used direct extrapolations i = 5 86 ⊕ 0 (Canup 2008), and (ii) assume Ω (Grießmeier 2006). from Earth's seismic model to integrate pressure and density f = 0 We compare these τ values with the stellar age of the along adiabatic profiles. These integrations provide radial sync host stars (for stars with no age estimates, we assume a lower density profiles for rocky exoplanets with an iron core, from age limit of 1 Gyr) (Table A1) and find that ∼99% of rocky which Zeng et al.(2016) proposed a semi-empirical relation- exoplanets in our sample are tidally locked. (τ < stellar ship between core mass fraction (CMF), planetary radius, sync 2π and mass. Zeng & Jacobsen(2017) then used the assump- age). For these we set Ω = orbital period in equation (3). tion that the internal gravity profile can be approximated as a piecewise function to show that the CMF can be re- latedp to the core radius fraction (CRF) of a rocky planet as CRF≈ CMF. The planetary core radius can then be calcu- lated as: v tu " 0:27# r 1 Rp Mp 0 = 1:07− / (2) Rp 0:21 R⊕ M⊕ where Rp and Mp are the planet's radius and mass respec- tively. In equation (1) F represents the strength of the ther- mal and chemical convection-driven dynamo in the planet's outer liquid core (L´opez-Morales et al. 2011). F is expressed Figure 1. Histogram of the rotation rates of 43 non-tidally locked in terms of the local Rossby number ROl , the thickness of solar system objects in units of Earth's rotation rate Ω⊕ (Ta- the outer liquid core D, and the rotation rate Ω, with all ble A2). The rotation rates of Jupiter and Saturn indicate that a three normalized to their corresponding Earth values: mass-weighted distribution of the 43 solar system objects would result in a similar mean with a narrower spread. We conserva- tively use this broader, number-weighted distribution. 2 2/3 7/3 F RO D Ω = l (3) F⊕ R D⊕ Ω⊕ Ol⊕ Since we do not have the planetary rotation rates for where D = 0:65r0 is the radial extent of the convection cell non-tidally locked exoplanets, we examine the rotation rate based on the Heimpel et al.(2005) model for the most effi- of 43 solar system objects including planets, moons, dwarf cient dynamo for at least some period of the planet's lifetime. planets, Kuiper belt objects and main-belt asteroids (Fig.1, We use a D = 0:65r0 conversion for all the rocky exoplanets Table A2). Their distribution is characterised by an average in our sample and D⊕ = 0:65r0⊕ for the outer liquid core rotation rate of Ω = 2:5 ± 1:5 Ω⊕ and we assign this value to radius of the Earth. Olson & Christensen(2006) calculated the five exoplanets in our sample that are not tidally locked.
Details
-
File Typepdf
-
Upload Time-
-
Content LanguagesEnglish
-
Upload UserAnonymous/Not logged-in
-
File Pages14 Page
-
File Size-