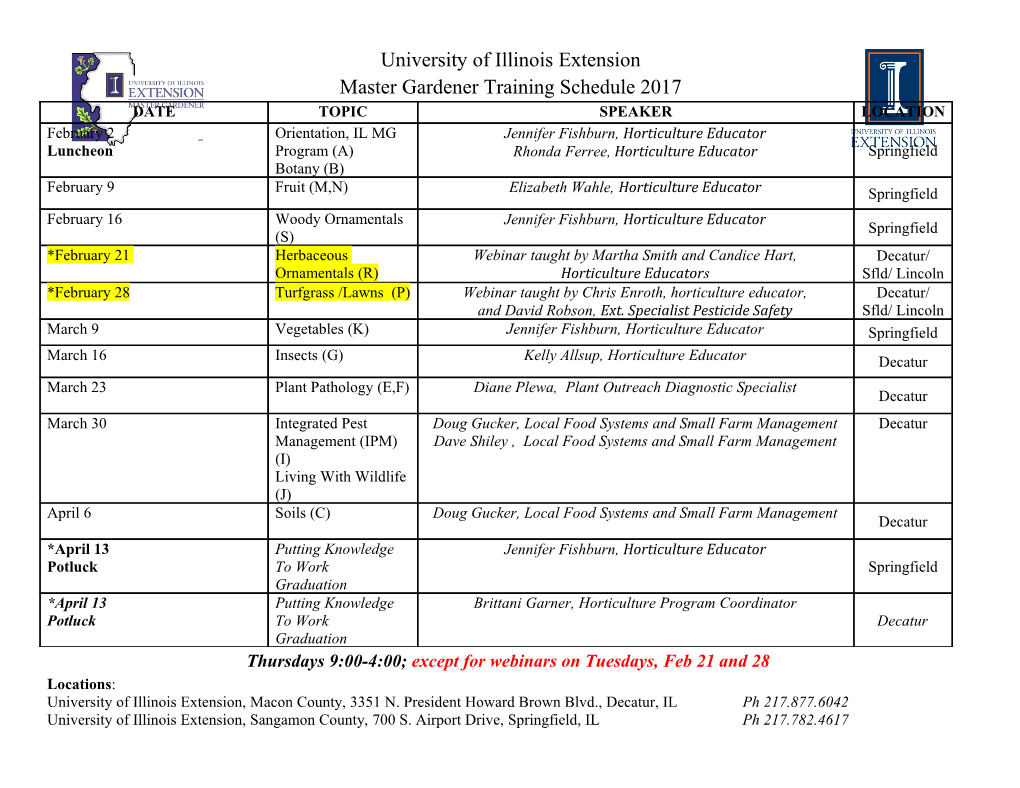
PASJ: Publ. Astron. Soc. Japan 51, 911-918 (1999) NANTEN Observations of Dense Cores in the Corona Australis Molecular Cloud Yoshinori YONEKURA Department of Earth and Life Sciences, Osaka Prefecture University, Sakai, Osaka 599-8531 E-mail (YY): [email protected] and Norikazu MIZUNO, Hiro SAITO, Akira MIZUNO, Hideo OGAWA* and Yasuo FUKUI Department of Astrophysics, Nagoya University, Chikusa-ku, Nagoya, ^64-8602 Downloaded from https://academic.oup.com/pasj/article/51/6/911/1467816 by guest on 30 September 2021 (Received 1999 August 26; accepted 1999 October 24) Abstract We carried out a C180 survey for dense molecular cores in the Corona Australis (CrA) molecular cloud with the NANTEN telescope. We observed 2.2 deg2 at a 2' grid spacing with a 2/7 beam, and 1980 positions were observed. We identified 8 C180 cores, whose typical line width, average column density, radius, mass, and average number density were 0.66 km s_1, 1.1 x 1022 cm-2, 0.13 pc, 18M®, and 1.4 x 104 cm-3, respectively. We found that AKomp, (Af(H2)), R, M, and n(H2) become larger along with an increase in the star-formation activity, whereas the ratio Mv-ir/M becomes smaller. A comparison of the present cores with those in Chamaeleon, Lupus, Ophiuchus, Taurus, and L 1333 indicates that star-forming cores tend to have a high column density, as well as a smaller MV-1T/M ratio. Key words: ISM: clouds — ISM: individual (Corona Australis Cloud) — ISM: molecules — stars: formation — radio lines: ISM 1. Introduction T CrA (FOe), TY CrA (B9e), and W CrA (Kl); there are also > 20 embedded IR sources, including the 'coro­ Recent observational studies have revealed that there net' cluster (Taylor, Storey 1984; Graham 1992; Wilking are a great variety of star-formation activities; only low- et al. 1992; Wilking et al. 1997). The brightest star in mass stars are born in dark clouds, whereas stellar groups the cloud is a Herbig Be star, TY CrA. These signa­ and massive stars are born in giant molecular clouds. tures of young stars make the CrA cloud one of the best One would expect that the star-forming activity in a regions to study intermediate-to-low-mass cluster forma­ cloud is correlated with the properties of the dense cores, tion along with the p Oph cloud. Although a number of since dense cores are the sites of present star formation. molecular line studies have been made toward the cloud Such a correlation, however, is still not observationally (Loren 1979; Loren et al. 1983; Levreault 1988; Harju et established, partly because of the lack of a rich sample al. 1993; Anderson et al. 1997), they are limited to the of dense cores. It is thus important to investigate the re­ densest part of the cloud, i.e., the R CrA cloud. lationship between the physical properties of dense cores We have carried out a survey for dense cores toward and the star-formation activities based on a rich sample the entire CrA cloud in ClsO (J = 1-0) emission at of dense cores in a systematic, statistical manner. 2.7 mm wavelength. In this paper, we present the physi­ The Corona Australis (CrA) molecular cloud is one cal properties of dense cores in the CrA molecular cloud of the nearest star-forming molecular clouds at 130 pc and compare the star-formation activity with the physi­ (Marraco, Rydgren 1981). The overall structure of the cal properties of dense cores. We also compare the char­ cloud has been investigated by studies of visual extinction acteristics of dense cores with those in the other nearby (Rossano 1978; Andreazza, Vilas-Boas 1996; Cambresy star-forming regions, such as Chamaeleon, Lupus, Ophi­ 1999). The cloud is highly elongated, having a dense uchus, Taurus, and L 1333. 'head' in the west (usually called the R CrA cloud) and a diffuse 'tail' in the east. Star formation is mainly taking 2. Observations place in the R CrA cloud. There are a number of pre- main-sequence stars, such as R CrA (A5e), S CrA (K6), C180 (J = 1-0) observations were made with the * Present address: Department of Earth and Life Sciences, Osaka NANTEN millimeter-wave telescope of Nagoya Univer­ Prefecture University, Sakai, Osaka 599-8531. sity at Las Campanas Observatory of Carnegie Institu- © Astronomical Society of Japan • Provided by the NASA Astrophysics Data System 912 Y. Yonekura et al. [Vol. 51, tion of Washington from 1998 February to March. The main-dish diameter of the NANTEN telescope is 4-m, providing a half-power beam width of 2/7 at 110 GHz, corresponding to ~ 1 pc for the distance of the CrA molecular cloud, 130 pc. The front-end was a 4 K cooled SIS mixer receiver (Ogawa et al. 1990). The typical sys­ tem temperature was 140 K (SSB) at 110 GHz, includ­ ing the atmosphere toward the zenith. The spectrometer was of the acousto-optical type with a total bandwidth of <D 40 MHz divided into 2048 channels. The effective spec­ <D k- tral resolution was 40 kHz, corresponding to a velocity O) <D resolution of 0.11 km s_1 at 110 GHz. The data were Downloaded from https://academic.oup.com/pasj/article/51/6/911/1467816 by guest on 30 September 2021 obtained with a grid spacing of 2' by using the frequency- •D switching technique with a switching interval of 13 MHz. The total integration time per point was ~ 1 min, and the !§ total number of observed points was 1980 (see figure 1). o The typical rms noise of the data was ATrms ~ 0.17 K at _1 CO a velocity resolution of 0.1 km s . To calibrate the spec­ CD tral line intensity, a room-temperature chopper wheel was employed. The absolute intensity calibration was made by observing p Oph East [a(1950) = 16h29m20.s9, 5(1950) = -24°22'13"] every 2 hours. The peak radi­ ation temperature, T^, of p Oph East was taken to be 4.4 K. A 3 a noise level of 0.24 K km s-1 was achieved in the final spectrum in the integrated intensity. The 1 0 pointing accuracy was measured to be better than 20". Galactic longitude (Degree) 3. Results and Discussion Fig. 1. Integrated intensity map of ClsO (J = 1-0) 18 shown in galactic coordinates. Equatorial coordi­ 3.1. Identification of C O Cores nates are also presented. The contour levels are 0.24 Figure 1 shows the total intensity distribution of the (3 a), 0.48, 0.96, 1.92, and 3.84 K km s"1. The dots ClsO (J = 1-0) spectra of the CrA molecular cloud. indicate the observed positions. We covered ~ 2.2 deg2, corresponding to ~ 11 pc2 at a distance of 130 pc. The map shows the existence of sev­ eral molecular condensations other than the main con­ of the ClsO cores are listed in table 1. densation, the R CrA cloud (Core 2, see table 1). In figure 2, we show a series of channel maps integrated 3.2. Physical Properties of Dense Cores over a velocity interval of 0.5 km s_1 in a velocity range -1 The physical properties of dense cores were estimated of 3.5 < FLSR < 7.5 km s . The velocity structure is in the following manner. For simplicity, we assumed the significant in Core 2; the westernmost part of Core 2 is _1 local thermodynamical equilibrium (LTE). We assumed ~ 1 km s redshifted from the rest of the cloud. This that the molecules along the line of sight possess a uni­ result is consistent with previous studies (Loren 1979; form excitation temperature, that the lines of the iso- Harju et al. 1993). topomers are at the same excitation temperature, and In order to study the physical properties of the dense 18 that the beam-filling factors are unity, although the less- regions, we define a C 0 core in the same manner as that abundant isotopes might be subthermally excited or their adopted by Onishi et al. (1996): (1) find a peak-intensity emission might arise primarily from the cloud interiors position, (2) draw a contour at a half level of the peak where the excitation conditions are different. In order intensity, (3) identify a core unless previously identified to estimate the excitation temperature, Tex, we observed cores exist within the half-level contour, (4) find the next the entire CrA cloud in 12CO (J = 1-0). Details of the intensity peak outside the core, (5) repeat the procedure 12CO observations will be published in a forthcoming pa­ after (2) until the peak intensity falls down below 6 a 1 per. We estimated Tex using the equation of radiative level (= 0.48 K km s" ). transfer for the optically thick line We finally identified 8 C180 cores. In figure 3, we show only the positions of the cores; the observed properties 5.53 (K), (1) ln{l + 5.53/[T£(12CO)(K) + 0.819]} © Astronomical Society of Japan • Provided by the NASA Astrophysics Data System No. 6] Dense Cores in the Corona Australis Cloud 913 Table 1. Observed properties of C180 cores in CrA. Position Core number 1 6 a(1950) (5(1950) TK VLSR AV 1 1 (°) (°) /h m s\ (° ' ") (K) (kms" ) (km s" ) 1 359.53 -20.47 19 10 15.0 -38 17 12 0.6 5.5 0.6 2 359.97 -17.80 18 58 20.3 -36 58 35 3.9 5.6 1.3 3 0.03 -18.93 19 03 45.9 -37 19 18 1.6 5.2 0.5 4 0.23 -18.77 19 03 18.9 -37 05 09 0.9 5.3 0.7 Downloaded from https://academic.oup.com/pasj/article/51/6/911/1467816 by guest on 30 September 2021 5 0.37 -19.50 19 06 59.1 -37 13 23 3.3 5.5 0.7 6 0.90 -20.43 19 12 14.7 -37 04 05 0.8 6.0 0.6 7 0.93 -20.17 19 11 02.3 -36 56 57 2.2 5.7 0.4 8 1.33 -20.50 19 13 14.2 -36 42 28 2.1 5.5 0.5 I 1 ' ' ' I ' ' ' ' I 1 1 1 L 3.5-4.0 kms" 4.0-4.5 kms"' 4.5-5.0 kms" 5.0—5.5 kms" -1.0 0.5 -0.0 Kkms"1 1.0 pc HPBW _L_ -17 1 ' ' I ' I 1 I • • 1 ' ' ' I ' ' ' ' I .
Details
-
File Typepdf
-
Upload Time-
-
Content LanguagesEnglish
-
Upload UserAnonymous/Not logged-in
-
File Pages8 Page
-
File Size-