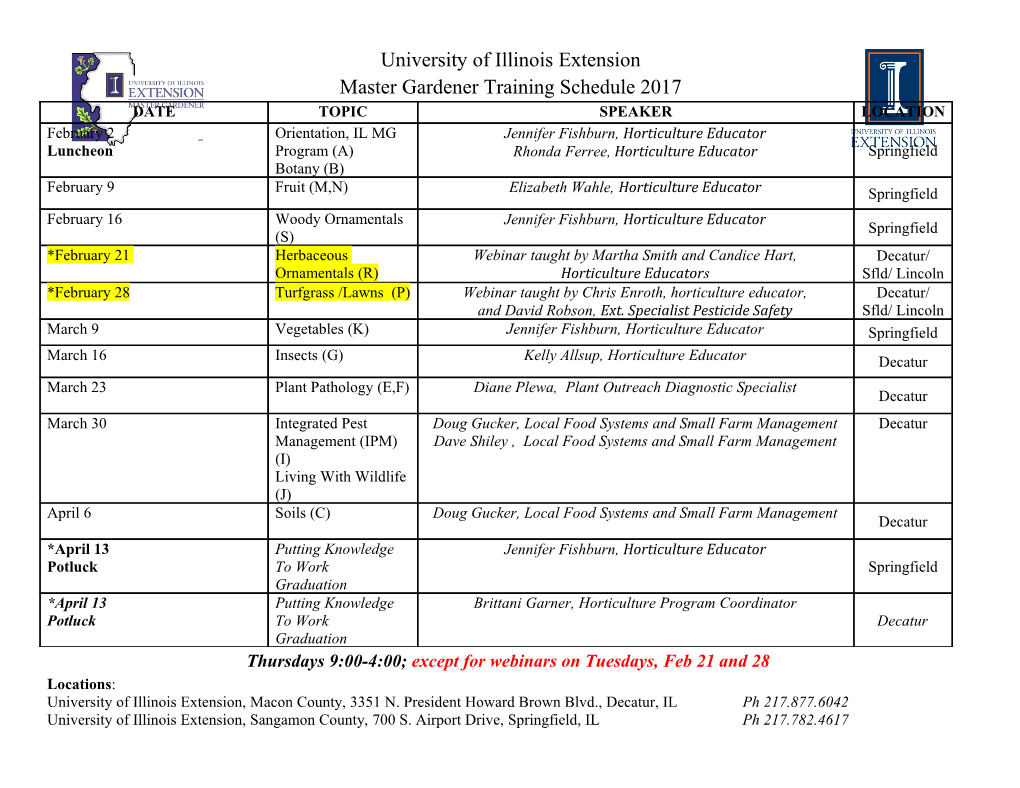
1 Observability of R-Hadrons at the LHC Andrea Rizzi, ETH Zuerich, CMS Collaboration Moriond QCD, 17-24/03/2007 O u t l i n e 2 ● Introduction to R-hadrons ● R-hadrons hadronic interactions ● LHC production ● Detector signature ● Trigger ● Slow particles mass measurement H e a v y S t a b l e C h a r g e d P a r t i c l e s 3 ● Some theories extending the SM predict new HSCP (hep-ph/0611040) – Gauge Mediated SuSY breaking => stau – Split Supersymmetry ● very high mass for scalars ● gluino is long lived – Other models: GDM, AMSB, extrDim, stable stop, 4th gen fermions ● To be clear: – heavy = mass in 100 GeV – few TeV region – stable = ctau > few meters (stable for LHC exp) ● LHC detectors are not designed for such particles I n t r o d u c t i o n t o R - h a d r o n s ● If new heavy stable particles are coloured they will form new 4 hadrons ● gluinos or stops are example of such particles ● New hadrons formed with new SuSY particles are called R-hadrons ● Several different states can be formed : Gluino: stop: baryons ~gqqq baryons ~tqq antibaryons ~g qbar qbar qbar antiparyons ~tbar qbar qbar mesons ~g q qbar mesons ~t qbar glue-balls ~g g antimesons ~tbar q ● Some are charged, some are neutral ● The charge can change in hadronic interactions with matter while crossing the detector (by “replacing” the light quarks bounded to the heavy parton) ● Simulation of hadronic interactions is crucial to understand LHC detectability H a d r o n i c i n t e r a c t i o n s ● Some models have been proposed to understand R-hadrons / matter interaction 5 ● The common starting points are (see Moriond QCD 2005 talk A.Kraan): – the heavy spectator is mainly a kinetic energy reservoir and does not actually take part in the interaction – the pion-nucleon interaction is used for cross section estimation (12mb per u/d quark and 6mb per s quark) [hep-ex/0404001] ● Results (energy loss per hadronic interaction) of different model are similar: hep-ph/0611040 hep-ph/0612161 Few GeV per interaction (tot Ekin > 100 GeV) => not showering in calorimeters A variant of the interaction model proposed in [hep-ex/0404001] has been implemented in Geant4 to allow LHC experiment simulations [hep-ph/0612161] H a d r o n i c I n t e r a c t i o n s I I An interesting effect is predicted for r-hadrons suffering several hadronic 6 interactions (e.g. crossing calorimeters): ● The probability that after an interaction an R-baryon, R-meson or R- antibaryon emerge depends on the heavy parton type ● Since matter is build of quarks we have gluino that the following processes are suppressed: stop – gluino-baryons to gluino-mesons antistop 1 6 – stop-baryons to stop-mesons 1 2 1 – 6 antistop-mesons to antistop-baryons 0 / h ● p The gluinos and the stop will tend to go to - p R-baryons while the antistop will go to R- e h meson ● Different energy release for baryon/mesons (different cross sections) => distinguish stop/antistop vs gluino L H C R - h a d r o n p r o d u c t i o n ● Up to now only stable gluino and stau scenarios have been fully simulated in LHC 7 experiment environment (stop hadrons are next) ● The fraction of neutral gluino-gluon ball is a free parameter (10% in our samples) ● The main process for gluino production at LHC gg->~g~g Cross sections @LHC: R.Mackeprang ~g Mass (GeV) Xsec 100 50nb 300 0,3nb 600 5pb 1000 0,1pb Velocity and momentum distributions at generator level D e t e c t o r s i g n a t u r e Summary of key features for LHC experiments: 8 ● Long lived particle (ct > few meters) ● Charged or neutral at production ● Suffering hadronic interaction (not showering) with ~1GeV energy loss per interaction (not stopped by calorimeters!) ● Low velocity: – high ionization – high time of flight ● Charge can change in every hadronic interaction – several interactions occur in calorimeters or other absorbers – charge in the inner tracking detector uncorrelated with charge in muon systems (outside calorimeters) – charge can change even inside the muon system C MS d e t e c t o r s i g n a t u r e 9 12.5ns late @ b =0.5 , h= 0 ● The signature is similar to the one of muons ● Standard Model and cosmic muons are the main backgrounds E f f e c t s o n d e t e c t a b i l i t y 10 Features that we can exploit: ● Muon like detector crossing -> muon trigger ● High time of flight can be measured -> mass reconstruction ● High ionization: dE/dX can be measured -> mass reconstruction ● Charge flip -> typical feature / distinguish from e.g. stable stau Experimental problems (detectors not designed for it): ● High time of flight -> out of bunch crossing (25ns) reconstruction ● High time of flight -> trigger time cuts ● High time of flight -> signal attenuation in tracker ● Neutral in inner tracker -> no muon/tracker tracks association ● Charge flipping in muon system -> lower reconstruction efficiency ● Charge flipping in muon system -> bad momentum measurement T r i g g e r ● Muon triggers are natural candidates. Typical 11 thresholds (from CMS) are: – single muon Pt > 37 GeV – single isolated muon Pt > 19 GeV – double isolated muon Pt > 7 GeV – additional constraint: delay wrt a muon Dt<12.5ns (i.e. beta >~0.6 for CMS) ● Muon calorimetric isolation can be an issue if hadronic interactions produce large calorimetric energy deposit ● Other triggers (jet, MET) can be used in model dependent/optimized analysis Main SM backgrounds are due to ● Muon only trigger are more model ●QCD (c/b -> muon + X) independent (gluinos, stop, stau, ....) ●W production ●Drell-Yan muons ● A final trigger efficiency ~ 15% is predicted ● with CMS full simulation for gluinos with top production M=600 GeV using standard triggers A n a l y s i s a n d m a s s m e a s u r e m e n t ● By reconstructing the mass the standard model backgrounds (high Pt 12 muon production) can be suppressed ● The mass is also a free parameter in theories predicting new stable charged particles ● Mass can be determined measuring velocity and momentum ● The velocity can be measured with two independent methods: – t.o.f. measurement in muon drift b-2 tubes – dE/dX measurement in tracker or calorimeters ● The momentum can be measured with inner tracker detector or with muon spectrometers – charge flipping makes CMS muon measurement not very reliable – neutral in tracker R-hadrons can be measured only in muon spectrometer B e t a a n d ma s s r e s o l u t i o n 13 ● Preliminary investigation in CMS Golden beta region – tracker dE/dX 0.6 < beta < 0.85 – Drift Tube time information ● best beta resolution (better than 5%) ● Full simulation (G4 model) used ● highest trigger efficiency ● Similar t.o.f. measurement techniques used for stable stau (GMSB) BETA (DT and Tk combined) MASS Ma s s p l o t 14 ● Backgrounds are removed by requiring low beta measurement both from dE/dX and from T.O.F. ● Different backgrounds for the two measurements ● Minimum Pt cut of 150 GeV is also applied 600 GeV L=30pb-1 L= 0.5 fb-1 C o n c l u s i o n s 15 ● LEP and Tevatron searches excluded stable gluinos up to ~300 GeV ● R-hadrons (as well as other Heavy Stable Charged Particles) can be observed at LHC exploiting their unique signature ● Trigger efficiency can still be optimized ● Mass measurement is possible using t.o.f. and ionization ● Detailed studies (reach with first data, etc..) going to be finalized this year ● Other models with similar signature can be studied in detail as well: – long lived stop – long lived stau – long lived KK states – long lived 4th generation fermions 16 BACKUP s - t a u v s r - h a d r o n s 17 MUONS STAU RHADRONS T r a c k e r d E / d X 18 100 GeV 600 GeV 300 GeV 3-4% beta resolution for beta in range [0.6-0.8] d E / d X c a l i b r a t i o n ( K & p ) 19 Calibration of module response can be obtained using K and / or protons S i g n a l a t t e n u a t i o n 20 TOB TEC TIB / TID Fraction of signal lost @beta=0.5 ●Too slow particle signal is attenuated because of the tracker electronics pulse shape. ●The dE/dX estimators weight more the lower signal hits (to cut Landau tails) ●Dedicated tool should be implemented to recover the effect D r i f t T u b e 21 ● R-hadron are late, so RecHit is further from wire than actual energy deposit Several ways to estimate tof: ● compute average distance of RecHit wrt reconstructed track (doesn't work if left and right hits are not the same number) ● make a fit where “tof” is a free parameter and hit position is function of “tof” (i.e. find the time of realignment of the hits) D r i f t t u b e r e s o l u t i o n 22 t.o.f.
Details
-
File Typepdf
-
Upload Time-
-
Content LanguagesEnglish
-
Upload UserAnonymous/Not logged-in
-
File Pages26 Page
-
File Size-