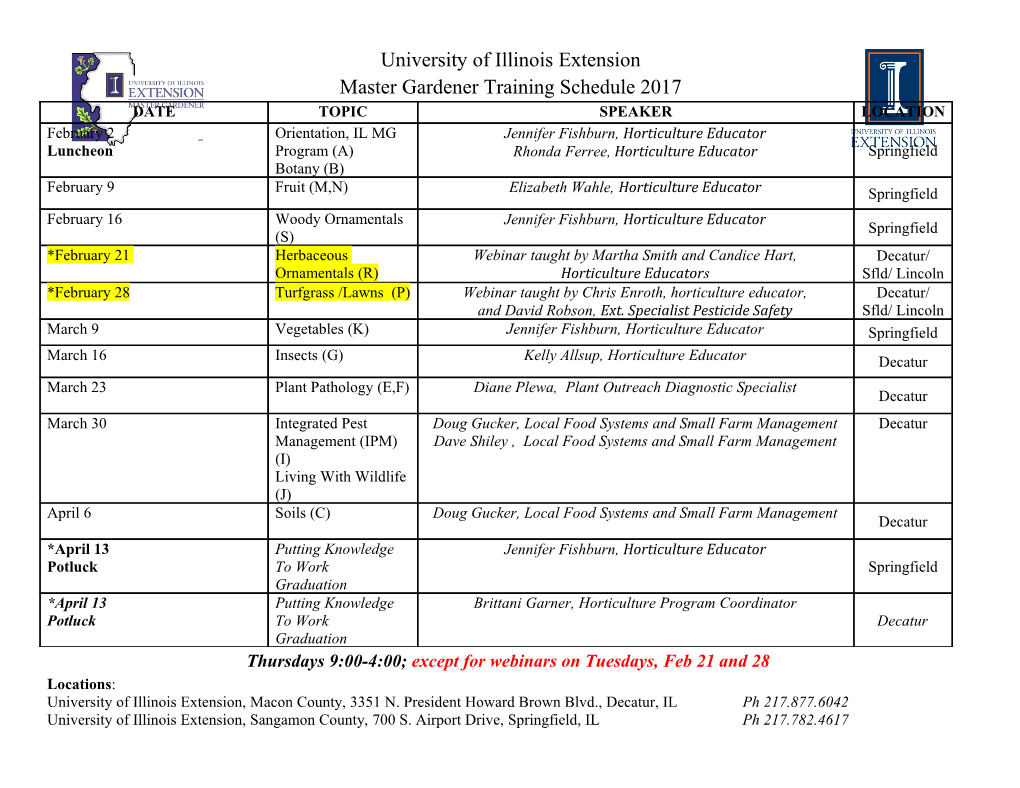
electric field. The mobility of ions is based on their size, Ion Mobility-Mass shape, and charge, thus IMS provides insights into struc- Spectrometry ture. In addition to being used for structural information, IMS can also be used as a separation device for complex mixtures. When coupled with mass spectrometry (MS), Wentao Jiang and Rena˜ A.S. Robinson IMS–MS offers a powerful hybrid analytical technique University of Pittsburgh, Pittsburgh, PA, USA that has many biological, pharmaceutical, structural, envi- ronmental, and other applications. This article provides an overview of IMS–MS, which focuses on principles 1 Introduction 1 of drift-time ion mobility spectrometry (DTIMS), high- 2 Principles of Ion Mobility Spectrometry 2 field-asymmetric ion mobility spectrometry (FAIMS), 2.1 Drift-Time Ion Mobility Spectrometry 2 and traveling wave ion mobility spectrometry (TWIMS) 2.2 High-Field-Asymmetric Waveform Ion methods. Several IMS–MS instruments are discussed and Mobility Spectrometry 4 examples of the current applications of the technology are 2.3 Traveling Wave Ion Mobility provided. Spectrometry 4 3 Ion Mobility Spectrometry–Mass Spectrometry Instrumentation 4 1 INTRODUCTION 3.1 Sources 5 3.2 Hybrid Instruments 6 IMS is a powerful analytical technique that has become 4 Multidimensional Ion Mobility more widespread in the last 40–50 years. IMS has several Spectrometry–Mass Spectrometry 10 capabilities as a stand-alone instrument and has been used (1–3) 4.1 Liquid Chromatography-Ion Mobility to monitor the detection of atmospheric compounds, (4–6) (7,8) Spectrometry-Mass Spectrometry 10 explosives, chemical warfare agents (CWAs), and (3) 4.2 Capillary Electrophoresis-Ion Mobility petrochemical reagents. In recent years, IMS tech- Spectrometry-Mass Spectrometry 11 nology has been used to detect explosives and narcotics in airport scanner devices. While it has been extremely 4.3 Ion Mobility Spectrometry-Ion Mobility effective in field applications as a stand-alone or portable Spectrometry-Mass Spectrometry 11 device, the coupling of IMS with MS extends the capabil- 4.4 Ion Mobility Spectrometry-Ion Mobility ities and applications of the technique tremendously. Spctrometry-Ion Mobility Spectrometry- IMS–MS is extremely useful for obtaining structural Mass Spectrometry 13 information on small polyatomic ions(9,10) to macromolec- 5 Applications of Ion Mobility ular ions, such as proteins(11–13) and even viruses.(12) Spectrometry–Mass Spectrometry 13 IMS–MS instruments can be operated in modes which 5.1 Proteomics 14 take advantage of IMS as a separation device allowing 5.2 Probing Structural Information 14 complex mixtures to be investigated and low-abundance 5.3 Lipidomics 14 species to be detected owing to the removal of chemical 5.4 Metabolomics 14 noise. Furthermore, IMS–MS provides fast measure- ments which allow it to be compatible with other front-end 5.5 Chiral Species 15 analytical separations, such as liquid chromatography and 5.6 Chemical Warfare Agents 15 capillary electrophoresis. 5.7 Pharmaceuticals 15 Owing to the growing interest in IMS–MS, this 5.8 Environmental 15 article seeks to provide a general overview of IMS–MS 6 Conclusions and Future Outlook 16 technology. There have been several notable advances in Acknowledgments 16 IMS–MS instrumentation which have led to a plethora of interesting applications. The reader will be introduced Abbreviations and Acronyms 16 to the basic principles surrounding three of the most Further Reading 16 commonly employed types of IMS separations. Current References 16 applications of IMS–MS have only been made possible due to the many advances that have taken place in instrumentation development and technology. Thus, an Ion mobility spectrometry (IMS) separates ions based on overview of several IMS–MS instrumentation setups is their mobility in an inert buffer gas in the presence of an also provided. Finally, examples of several applications Encyclopedia of Analytical Chemistry, Online © 2006–2013 John Wiley & Sons, Ltd. This article is © 2013 John Wiley & Sons, Ltd. This article was published in the Encyclopedia of Analytical Chemistry in 2013 by John Wiley & Sons, Ltd. DOI: 10.1002/9780470027318.a9292 2 MASS SPECTROMETRY that stem from IMS–MS are discussed and this article In the above expression, ze refers to the charge on concludes with a future outlook on potential applications the ion, kB is Boltzmann’s constant, mI and mB are the and advancements of IMS–MS. masses of the ion and buffer gas, respectively, and N is the number density of the buffer gas.(14) Because IMS can be coupled with MS the mass and charge of an ion can 2 PRINCIPLES OF ION MOBILITY be readily deduced. By operating at specific fields (i.e. SPECTROMETRY low or high) or with different pressure regimes, different IMS methods can be developed. Here we discuss three The basic principle of ion mobility separation can common approaches: DTIMS, FAIMS, and TWIMS. be simply described as a gas-phase electrophoresis technique, whereby gaseous ions are separated according to their size, shape, and charge in the presence of a 2.1 Drift-Time Ion Mobility Spectrometry weak electric field. The drift tube is filled with an inert buffer gas (i.e. argon, helium, and nitrogen) at either low DTIMS–MS is the most widespread developed and vacuum pressures or at atmospheric pressure conditions. employed approach. DTIMS is the only IMS method Ions move according to diffusion processes through the which provides a direct measure of collision cross-section drift tube as the energies of the ions are similar to the based on an ion’s mobility. Figure 1(a) shows a simple thermal energy of the buffer gas. Various ions will have drift tube instrument that is filled with inert buffer gas different mobilities in a given drift tube device which in a counter direction of the ion motion. The weak elec- allows the separation of mixtures of ions and structural tric field applied to the drift tube is generated using information to be obtained. The simplest configuration a series of resistors and a DC potential. The electric of a drift tube is one in which a series of stacked ring field applied is generally around 2.5–20 V cm−1(15,16) in electrodes have a static direct current (DC) field applied reduced-pressure IMS (i.e. the drift pressure ranges from across the electrodes and the tube is filled with an inert 1 to 15 mbar). Higher voltages are applied across the drift buffer gas. As ions move under the influence of this tube when higher pressures, such as atmospheric pres- ν weakly applied electric field, they have a velocity, D, sures, are used.(17,18) Regardless of the pressure regime which is governed by the electric field, E, and mobility of used, it is important that the voltages applied do not cause the ion, K, in a specific buffer gas. the potential breakdown of the buffer gas. Traditionally used buffer gases are helium, nitrogen, and argon, or ν = KE (1) D mixtures thereof.(19–24) K is measured experimentally based on the time it takes DTIMS does not work with continuous injection of an ion to traverse the drift tube of length, L. ions, therefore packets of ions are introduced into the drift tube using an ion gate(17,25) or ion funnel.(15,26) Ion L packets can range in width from 100 to 200 μs. Because of K = ( ) 2 the use of ion packets, the overall sensitivity of the method tDE is reduced(27) such that only 0.1–1% of ions generated are Comparisons of reduced ion mobilities, K0, across sent to the IMS. After the ions are injected into the drift laboratories can be obtained by normalizing for buffer tube, the species begin to separate based on their mobility gas pressure, P , and temperature, T , as follows: through the buffer gas. For example, doubly-charged species experience the force of the electric field twice as L 273 P = much as singly-charged ions, therefore for ions of the same K0 (3) EtD T 760 shape the doubly-charged ion will have a higher mobility through the tube and thus a shorter drift time. Also, ions It is often useful to deduce information about the which have more elongated conformations will undergo structure (i.e. the size and shape) of specific ions based more collisions with buffer gas atoms and thus take a on a mobility experiment. This is possible using an experimentally derived collision cross-section, , for an longer time to drift through the tube than more compact ion, which represents the average area of the molecule structures. These concepts are illustrated in Figure 1(a). that interacts with the buffer gas over a range of three- Typically, ions travel through the drift tube on the (28) dimensional orientations. order of milliseconds which makes for a relatively fast separation. As can be inferred from the mobility (18π)1/2 ze 1 1 1/2 t E 760 T 1 equations described, the length of the drift tube can = + D 1/2 influence the transient time and mobility of an ion. The 16 (kBT) mI mB L P 273 N (4) drift resolving power (t/t) at full-width half maximum Encyclopedia of Analytical Chemistry, Online © 2006–2013 John Wiley & Sons, Ltd. This article is © 2013 John Wiley & Sons, Ltd. This article was published in the Encyclopedia of Analytical Chemistry in 2013 by John Wiley & Sons, Ltd. DOI: 10.1002/9780470027318.a9292 ION MOBILITY-MASS SPECTROMETRY 3 Electric field (a) Drift gas Ion source Intensity Drift time Drift tube (b) ~V(t) Carrier CV1 gas CV2 Ion Intensity CV3 source CV CV2 CV (c) Drift gas Ion source Intensity t1 t2 Drift time Time tn Traveling wave potential Figure 1 Illustration of drift tube separation principles.
Details
-
File Typepdf
-
Upload Time-
-
Content LanguagesEnglish
-
Upload UserAnonymous/Not logged-in
-
File Pages21 Page
-
File Size-