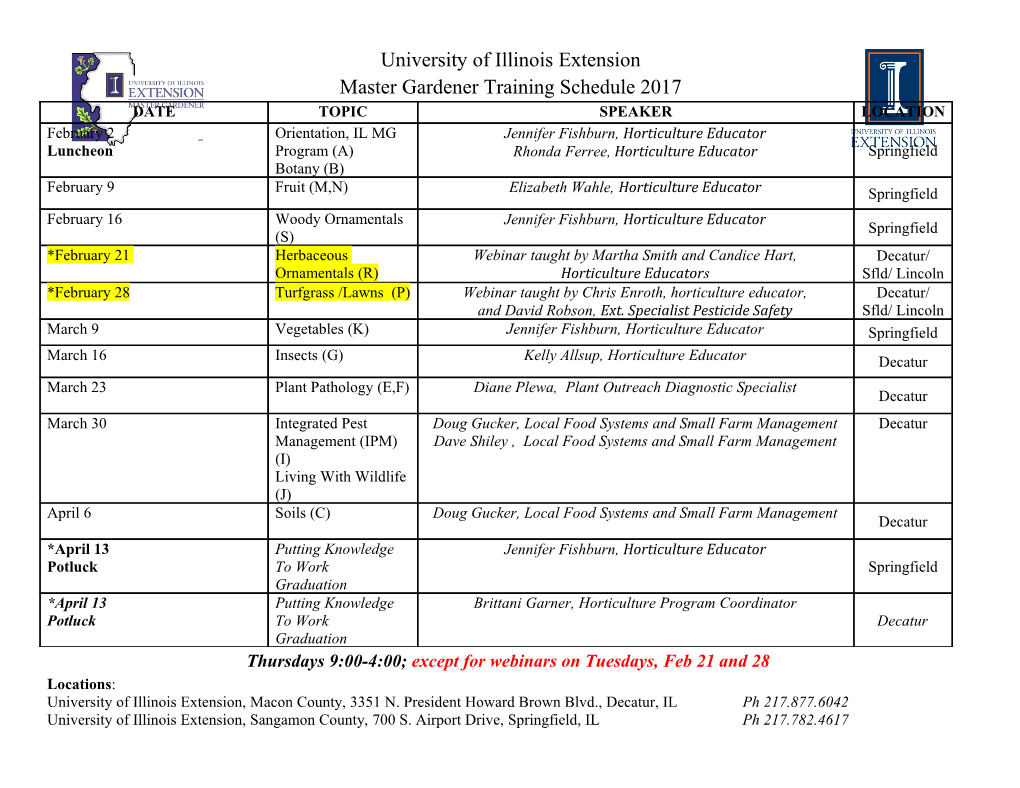
Advanced Physical Techniques in Inorganic Chemistry: Probing Small Molecule Activation The Harvard community has made this article openly available. Please share how this access benefits you. Your story matters Citation Anderson, Bryce L. 2016. Advanced Physical Techniques in Inorganic Chemistry: Probing Small Molecule Activation. Doctoral dissertation, Harvard University, Graduate School of Arts & Sciences. Citable link http://nrs.harvard.edu/urn-3:HUL.InstRepos:33493292 Terms of Use This article was downloaded from Harvard University’s DASH repository, and is made available under the terms and conditions applicable to Other Posted Material, as set forth at http:// nrs.harvard.edu/urn-3:HUL.InstRepos:dash.current.terms-of- use#LAA Advanced Physical Techniques in Inorganic Chemistry: Probing Small Molecule Activation A dissertation presented by Bryce L. Anderson To The Department of Chemistry and Chemical Biology in partial fulfillment of the requirements for the degree of Doctor of Philosophy in the subject of Chemistry Harvard University Cambridge, Massachusetts May 2016 © 2016 Bryce L. Anderson All rights reserved Dissertation Advisor: Professor Daniel G. Nocera Bryce L. Anderson Advanced Physical Techniques in Inorganic Chemistry: Probing Small Molecule Activation Abstract Robust and efficient catalysts are necessary for realizing chemical energy storage as a solution for the intermittency associated with renewable energy sources. To aid in the development of such catalysts, physical methods are used to probe the photochemistry of small molecule activation in the context of solar-to-fuels cycles. Three systems are studied in the context of HX splitting (X=Br, Cl): polypyridyl nickel complexes, NiX3(LL) (LL = bidentate phosphine) complexes, and dirhodium phosphazane complexes. Two systems are studied in the context of dioxygen activation: cryptand-encapsulated peroxide dianion and cubane models of cobalt water oxidation catalysts. Nickel complexes are shown to facilitate photo-driven H2 evolution from solutions containing HCl. Transient absorption (TA) reveals that polypyridyl ligands act as redox mediators, circumventing the inherently short excited state lifetimes common to first row transition metal complexes. By coupling the one-electron photochemistry of the polypyridyl ligands to disproportionation of reduced nickel complexes, two-electron chemistry is achieved. Halogen photoelimination is studied in a series of NiX3(LL) complexes which eliminate halogen in both solution and the solid state. Computation shows that efficient halogen iii photoelimination is facilitated by a dissociative LMCT excited state. TA identifies an aryl- halide complex as an intermediate in the photoelimination reaction. Halogen photoelimination from valence isomeric dirhodium phosphazane complexes, Rh2[I,III] and Rh2[II,II], is studied using TA and photocrystallography. TA suggests a common photo-intermediate that is probed by photocrystallography to reveal structural changes associated with transition to the proposed common intermediate. Oxygen activation chemistry is studied using the first soluble form of peroxide dianion. The kinetics of peroxide dianion oxidation are studied by leveraging the dianion’s propensity to form ion pairs with ruthenium polypyridyl complexes. TA kinetic data and DFT calculations facilitate a Marcus analysis which shows that the O–O bond of the peroxide dominates the internal reorganization energy. Cubane model complexes structurally related to the CoPi water oxidation catalyst are studied using TA and computations. Photooxidation by a covalently linked photosensitizer exhibits fast electron transfer rates. Calculations show extensive delocalization of the cubane molecular orbitals and examination of the excited state manifold implicates d–d excited states as facilitators of rapid charge transfer. iv Table of Contents Title Page i Copyright Page ii Abstract iii Table of Contents v List of Figures viii List of Tables xviii List of Abbreviations xix Acknowledgements xxi Chapter 1. Introduction 1 1.1. The Growing Global Demand for Energy 2 1.2. Solar Energy 4 1.3. The Challenges of Splitting H2O 6 1.4. HX (X=Cl, Br) as an Alternative to H2O for Solar Energy Storage 10 1.5. Photochemical Solar-to-Fuels Cycles 11 1.5.1. Photochemical Hydrogen Production 12 1.5.2. Photochemical HX Splitting (X = Br, Cl) 14 1.5.3. Photochemical Water Oxidation 15 1.6. Probing Small Molecule Activation 18 1.6.1. Transient Absorption 18 1.6.2. Photocrystallography 20 1.7. Scope of Thesis 23 1.8. References 26 Chapter 2. Spectroscopic Observation of Intermediates in an HCl to H2 Photocycle Promoted by 38 Ni(II) Polypyridyl Halide Complexes 2.1. Introduction 39 2.2. Results 40 2.2.1. Steady-State Photochemistry 40 2.2.2. Thermal Proton Reduction Reactions 45 2.2.3. Time-Resolved Photochemical Experiments 47 2.3. Discussion 52 2.4. Conclusion 54 2.5. Experimental 56 2.6. References 65 v Chapter 3. Spectroscopic Observation of Secondary 68 Coordination Sphere Effects in Halogen Photoelimination 3.1. Introduction 69 3.2. Results 72 3.2.1. Characterization of Mononuclear Ni(III) Complexes 72 3.2.2. Halogen Photoelimination Chemistry 76 3.2.3. Time-Resolved Photochemistry 81 3.2.4. Solution-Phase Calorimetry 92 3.2.5. Computational Results 95 3.3. Discussion 102 3.4. Conclusion 105 3.5. Experimental 106 3.6. References 114 Chapter 4. Observation of Chloride-Bridged 120 Photointermediates in Valence-Isomeric Rh2 Complexes 4.1. Introduction 121 4.2. Results 123 4.2.1. Steady State Photochemistry of Dirhodium Isocyanides 123 4.2.2. Transient Absorption Spectroscopy of Dirhodium Isocyanides 125 4.2.3. Photocrystallography 128 4.2.4. Electronic Structure Calculations 132 4.2.5. Photochemistry of Dirhodium Carbonyls 136 4.3. Discussion 139 4.4. Conclusion 143 4.5. Experimental 144 4.6. References 156 Chapter 5. Charge Transfer Studies of Peroxide Dianion 161 5.1. Introduction 162 5.2. Results 164 5.2.1. Ion Pair Formation 164 5.2.2. Stern-Volmer Emission Quenching 165 5.2.3. Nanosecond Transient Absorption 167 5.2.4. Picosecond Transient Absorption 169 5.2.5. Marcus Analysis of Unimolecular ET Rate Constants 174 5.2.6. Evaluation of the Internal Reorganization Energy 177 5.3. Discussion 180 5.4. Conclusion 182 5.5. Experimental 183 5.6. References 198 vi Chapter 6. Electron Transfer in Cobalt Cubane WOC Model 202 Systems 6.1. Introduction 203 6.2. Results 206 6.2.1. Photosensitization of the Co4O4 Model Systems 206 6.2.2. Covalent Attachment of NMI to the Cubane Models 208 6.2.3. Thermodynamics of Photoinduced Charge Transfer 210 6.2.4. Ultrafast Photooxidation of Co4O4 Model Systems 213 6.2.5. Second Oxidation of the Cobalt Cubanes 217 6.2.6. DFT Calculation of the Cubane Excited State Manifold 221 6.3. Discussion 224 6.4. Conclusion 227 6.5. Experimental 228 6.6. References 246 vii List of Figures Figure 1.1. (a) Historical and predicted total world energy consumption from 1990 2 through 2040, (b) world energy consumption by fuel type. Adapted from International Energy Outlook 2013: U.S. Energy Information Administration. Figure 1.2. Atmospheric CO2 concentrations measured at Mauna Loa Observatory, 3 Hawaii. Dr. Pieter Tans, NOAA/ESRL (www.esrl.noaa.gov/gmd/ccgg/trends/) and Dr. Ralph Keeling, Scripps Institution of Oceanography (scrippsco2.ucsd.edu/). Figure 1.3. Cumulative US generation capacity from renewable sources in GW. (a) The 4 growth of generation from hydropower has been stagnant while other renewable sources have seen significant growth. (b) Solar and wind power compose the bulk of the growth of renewable energy sources. Adapted from Sustainable Energy in America 2016 Factbook: Bloomberg New Energy Finance and The Business Council for Sustainable Energy (Copyright 2016) Figure 1.4. Energy and power densities of various energy storage solutions. Chemical 5 energy storage dominates the high energy density region. Reprinted with permission from Cook et al. Chem. Rev. 2010, 110 (11), 6474–6502. Copyright 2010 American Chemical Society. Figure 1.5. Oxygen Latimer diagram. High energy intermediates are highlighted in red. 7 Figure 1.6. Potential mechanisms of O–O bond formation promoted by terminal metal- 7 oxo complexes. Figure 1.7. The Mn4CaO5 cubane structure of the active site of the PSII oxygen evolving 9 complex. Figure 1.8. Three component system utilizing a sacrificial reductant as a substitute for 12 – – the anodic reaction. In principle, component D can represent H2O/OH , X , or any other source of electrons. Figure 1.9. Pt2(III,III) provides the first example of trap free halogen photoelimination. 15 In the solid state Cl2 can be captured under vacuum to demonstrate authentic halogen elimination. Figure 1.10. The Rubpy/persulfate flash quench reaction is used to photochemically 16 oxidize water to O2. Figure 1.11. Potential H2O splitting cycle mediated by a ruthenium (II) pincer complex 17 presented by Milstein and coworkers. viii Figure 1.12. Diagram of typical TA experimental configurations. (a) Nanosecond TA 19 often consists of a continuous probe source and a pulsed laser, commonly a Nd:YAG. Timing is performed electronically using a gated CCD camera or recorded on a ADC, typically an oscilloscope. (b) Picosecond and Femtosecond experimental configuration consists of a time-of-flight delay scheme controlled by a translation stage. Both pump and probe are pulses generated from the same Ti:Sapphire oscillator. Figure 1.13. Diffraction pattern obtained from Fluorspar crystals and the predicted 21 diffraction pattern. Figures adapted with permission from Bragg, W. L. The Structure of Some Crystals as Indicated by Their Diffraction of X-Rays. Proceedings of the Royal Society of London A: Mathematical, Physical and Engineering Sciences 1913, 89 (610), 248–277. Figure 1.14. Typical photocrystallography experimental apparatus. If the experiment is 22 using continuous irradiation the X-ray chopper is absent or stopped in the ‘open’ position. Figure 2.1. Spectral evolution during photolysis of (a) Ni(II) complex 1 to Ni(0) complex 41 2 in THF and (b) Ni(II) complex 1 to Ni(I) complex [3]Cl in 1:1 CH3CN:1,4– cyclohexadiene.
Details
-
File Typepdf
-
Upload Time-
-
Content LanguagesEnglish
-
Upload UserAnonymous/Not logged-in
-
File Pages275 Page
-
File Size-