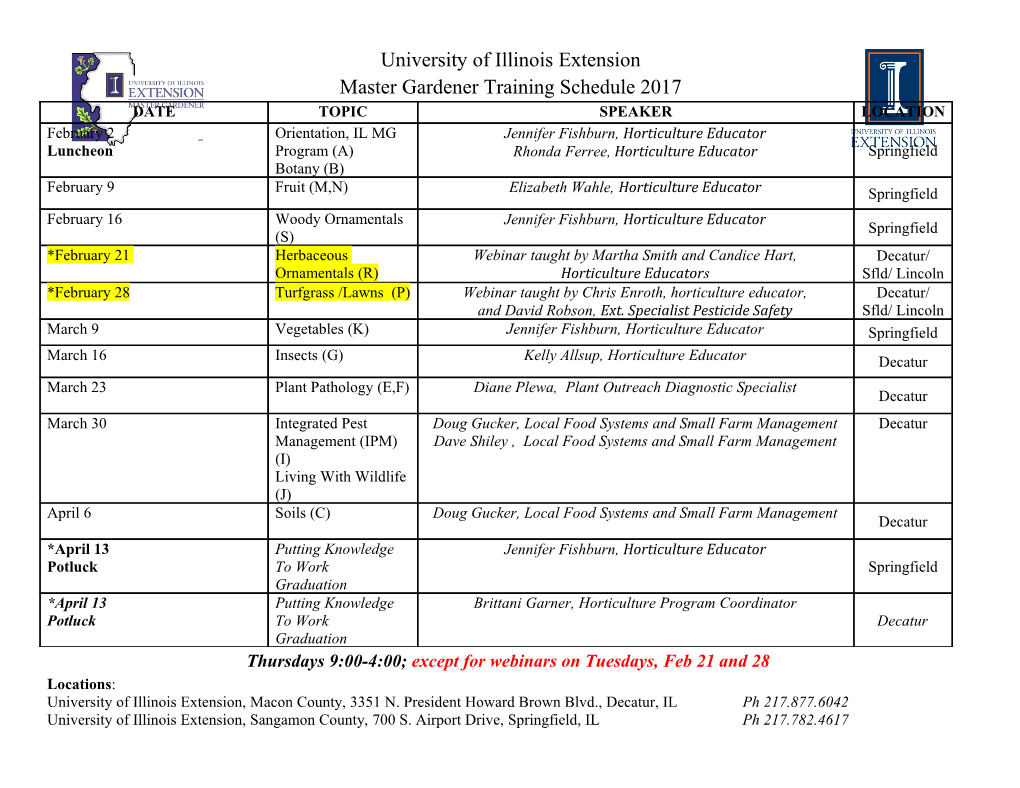
Structural basis of light-induced redox regulation in the Calvin–Benson cycle in cyanobacteria Ciaran R. McFarlanea,1, Nita R. Shaha,1, Burak V. Kabasakala,2, Blanca Echeverriaa, Charles A. R. Cottona,3, Doryen Bubecka,4, and James W. Murraya,4 aDepartment of Life Sciences, Imperial College London, SW7 2AZ, United Kingdom Edited by Bob B. Buchanan, University of California, Berkeley, CA, and approved September 12, 2019 (received for review April 18, 2019) Plants, algae, and cyanobacteria fix carbon dioxide to organic ordering the C-terminal domain (16), enabling it to bind GAPDH, carbon with the Calvin–Benson (CB) cycle. Phosphoribulokinase which is then inhibited. (PRK) and glyceraldehyde 3-phosphate dehydrogenase (GAPDH) The GAPDH-CP12 complex can then bind PRK, in an obli- are essential CB-cycle enzymes that control substrate availability gate sequential reaction (17). Previous structural studies of the for the carboxylation enzyme Rubisco. PRK consumes ATP to pro- GAPDH-CP12 complex resolved only a C-terminal fragment of duce the Rubisco substrate ribulose bisphosphate (RuBP). GAPDH CP12, so it remained unclear how CP12 regulated both GAPDH catalyzes the reduction step of the CB cycle with NADPH to pro- and PRK. A recent structure of a cyanobacterial CP12-CBS 2 duce the sugar glyceraldehyde 3-phosphate (GAP), which is used domain protein resolved an N-terminal CP12-like region; how- for regeneration of RuBP and is the main exit point of the cycle. ever, the protein does not form a ternary complex with GAPDH GAPDH and PRK are coregulated by the redox state of a condition- and PRK (8). Calvin cycle GAPDH uses NADPH physiologically ally disordered protein CP12, which forms a ternary complex with to reduce BPG, although NADH may also be a substrate. both enzymes. However, the structural basis of CB-cycle regulation CP12 is conserved in oxygenic phototrophs from cyanobacteria by CP12 is unknown. Here, we show how CP12 modulates the to plants (18). Plants often have more than 1 CP12 isoform, with activity of both GAPDH and PRK. Using thermophilic cyanobacte- tissue-specific patterns of expression (19). Plants also have rial homologs, we solve crystal structures of GAPDH with different 2 photosynthetic GAPDH isoforms GAP-A and GAP-B. GAP-A cofactors and CP12 bound, and the ternary GAPDH-CP12-PRK com- is similar to the cyanobacterial enzyme while GAP-B has a BIOCHEMISTRY plex by electron cryo-microscopy, we reveal that formation of the C-terminal extension homologous to the C-terminal GAPDH N-terminal disulfide preorders CP12 prior to binding the PRK active binding domain of CP12 (20). GAP-A and GAP-B form an A2B2 site, which is resolved in complex with CP12. We find that CP12 tetramer, which further aggregates to A8B8 on oxidation of the binding to GAPDH influences substrate accessibility of all GAPDH GAP-B CP12-like region (20). Information from cyanobacteria is active sites in the binary and ternary inhibited complexes. Our still applicable to plants, as the cyanobacterial CP12 system is structural and biochemical data explain how CP12 integrates re- a subset of the plant regulatory system. The lack of structural sponses from both redox state and nicotinamide dinucleotide availability to regulate carbon fixation. Significance redox regulation | carbon fixation | photosynthesis | Calvin–Benson cycle The Calvin–Benson (CB) cycle in plants, algae, and cyanobac- teria fixes most of the carbon in most of the biomass on Earth. lants regulate their carbon fixation and other reactions by The CB cycle is regulated by the redox state, which enables it to Predox state using it as a proxy for light (1). The product of the be turned off in the dark. One part of this regulatory system is photosynthetic electron transport chain is reduced ferredoxin, the small protein CP12, which binds to 2 essential CB-cycle which is used to produce NADPH in the cyanobacterial cyto- enzymes in the dark, inactivating them. We have solved the plasm or chloroplast stroma. In the light, the chloroplast stroma structure of the complex between CP12 and the enzymes, is reducing, and in the dark, or when the light reactions are explaining the mechanism of deactivation. Now that this is otherwise inactive, the stroma becomes oxidizing. Thioredoxin understood, this structure can be used as the starting point for (2) proteins are reduced by ferredoxin and can then exchange a modulating the redox regulation, which may have applications disulfide with a target. Example targets are the sedoheptulose (3) in improving crop productivity. and fructose bisphosphatases (4), which have pairs of cysteines that oxidize to form disulfide bonds that inactivate the enzyme. Author contributions: C.R.M., D.B., and J.W.M. designed research; C.R.M., N.R.S., B.V.K., The carbon-fixation reaction is catalyzed by Rubisco, which B.E., C.A.R.C., D.B., and J.W.M. performed research; C.R.M., N.R.S., D.B., and J.W.M. ana- carboxylates ribulose bisphosphate (RuBP). RuBP is produced lyzed data; and C.R.M., N.R.S., D.B., and J.W.M. wrote the paper. by phosphoribulokinase (PRK) in the final regeneration reaction, The authors declare no competing interest. in which a phosphate group is transferred from ATP to ribulose 5- This article is a PNAS Direct Submission. phosphate (5). Plant-type PRK is dimeric and has 2 conserved This open access article is distributed under Creative Commons Attribution-NonCommercial- disulfide bonds, one at the N terminus and the other near the C NoDerivatives License 4.0 (CC BY-NC-ND). terminus close to the dimer interface (6, 7). The N-terminal disulfide Data deposition: Crystallography, atomic coordinates, and structure factors have been – deposited in the PDB and EMDB repositories: PDB IDs 6GFP, 6GFQ, 6GFR, 6GFO, 6GG7, bond inactivates most of the activity when formed (8 10), but may 6GHR, 6GHL, and 6GVE; EMDB ID EMD0071. be reduced in plants by thioredoxin f (11). 1C.R.M. and N.R.S. contributed equally to this work. The reduction step of the Calvin–Benson (CB) cycle is cata- 2Present address: School of Biochemistry, University of Bristol, BS8 1TD Bristol, lyzed by glyceraldehyde 3-phosphate dehydrogenase (GAPDH), United Kingdom. which uses NADPH to reduce bisphosphoglycerate (BPG) to 3Present address: Max Planck Institute of Molecular Plant Physiology, 14476 Potsdam- glyceraldehyde 3-phosphate (GAP), the main product exit point Golm, Germany. of the cycle (12). In cyanobacteria, GAPDH has no disulfide 4To whom correspondence may be addressed. Email: [email protected] or j.w. bonds, instead redox regulation of GAPDH is driven by 2 disulfide [email protected]. bonds in the small (∼8 kDa) regulatory inhibitor protein CP12 This article contains supporting information online at www.pnas.org/lookup/suppl/doi:10. (13, 14), which is disordered under reducing conditions (15). 1073/pnas.1906722116/-/DCSupplemental. Under oxidizing conditions, 2 disulfide bonds form in CP12, www.pnas.org/cgi/doi/10.1073/pnas.1906722116 PNAS Latest Articles | 1of7 Downloaded by guest on September 28, 2021 information for full-length CP12 and CP12-bound regulatory CP12 binding to GAPDH mutually exclusive. CP12-Glu69 is not complexes has prevented understanding of a key mechanism of only important for dinucleotide selection, but is also essential for CB-cycle redox regulation. To understand, at a molecular level, ternary complex formation in Chlamydomonas (24). how the CB cycle is redox regulated in response to light, we solved crystal structures of a thermophilic cyanobacterial GAPDH with Structure of Full-Length CP12 Bound to GAPDH. In 3 conditions, full-length CP12 and built an atomic model of the entire cyano- GAPDH-CP12 crystallized with one or more full-length CP12 bacterial GAPDH-CP12-PRK ternary complex using electron visible in the electron density, at a best resolution of 2.1 Å. We cryo-microscopy (cryoEM). obtained 4 crystallographically independent full-length oxidized CP12 molecules bound to GAPDH. CP12 comprises an N-terminal Results and Discussion PRK binding domain (residues 1–52) and a C-terminal GAPDH – Structures of GAPDH with Different Nucleotides Bound and CP12 binding domain (residues 55 75) connected by a fully flexible C-Terminal Regions. A previous structure of cyanobacterial linker (Fig. 2). If the C terminus of CP12 is bound to GAPDH, GAPDH bound to CP12 had 4 CP12 per GAPDH tetramer (21), the N-terminal domain can adopt any relative angle. Although but the stoichiometry for eukaryotic complexes suggested by the relative orientations of the N- and C-terminal domains of structure (22) and biochemistry (23) was 2. Working with recombinant CP12 vary in our structures, the structures of both domains are conserved across 4 crystallographically independent CP12 mol- proteins from the thermophilic cyanobacterium Thermosynechococcus ecules (Fig. 2 C and D). elongatus, we found the cyanobacterial GAPDH -CP12 complex was 4 2 The CP12 N-terminal PRK binding domain is a 2-helix bundle, stable to gel-filtration and crystallized with this stoichiometry. We stabilized by a disulfide bridge between Cys-19 and 29 near the call the 2 CP12-occupied active sites proximal and the 2 un- helical turn. The 2 helices bury a small hydrophobic core, including occupied sites distal. In all our structures, and those published, the a short leucine zipper. Helix-2 (27-52) contains the conserved 2 proximal sites are in equivalent positions in the GAPDH tet- CP12 characteristic motif, AWDA(V/L)EEL (Figs. 2B and 3E) ramer. We obtained a GAPDH4-CP124 structure when GAPDH (25), which forms an acidic patch on the surface. was incubated with 10-fold excess CP12 (SI Appendix,Fig.S1), so the physiological GAPDH-CP12 complex was unclear (21, 22). Structure of GAPDH-CP12-PRK Complex. We solved the structure of Only the C terminus of CP12 was visible in these structures.
Details
-
File Typepdf
-
Upload Time-
-
Content LanguagesEnglish
-
Upload UserAnonymous/Not logged-in
-
File Pages7 Page
-
File Size-