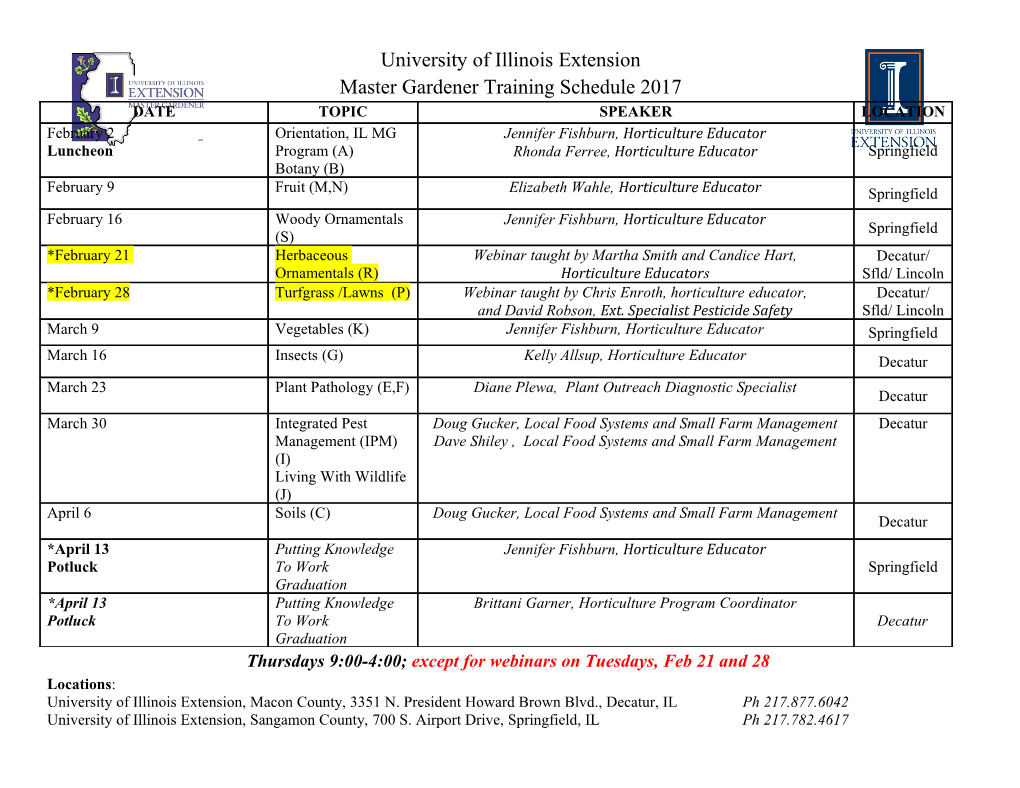
Measures of Membrane Fluidity: Melting Temperature • Tm (melting temperature) is a phase transition, a change from a more rigid solid-like state to a fluid-like state The fluidity - ease with which lipids move in the plane of the bilayer - of cell membranes has to be precisely regulated because many biological processes (e.g. membrane transport and some enzyme activities) cease when the bilayer fluidity is reduced too much. The fluidity of cell membranes depends on their chemical composition and also on temperature. As the temperature is raised, a synthetic bilayer made of one type of phospholipid undergoes a phase transition from a solid-like state to a more fluid-like state at a characteristic melting point temperature (abbreviated as Tm). At low temperatures the lipids within the bilayer are well- ordered, packed into a crystal-like arrangement in which the lipids are not very mobile and there are many stabilizing interactions. As the temperature is raised, these interactions are weakened, and the lipids are in a less ordered, liquid-like state. Lipids with longer fatty acid chains have more interactions between the hydrophobic fatty acid tails (predominantly van der Waals interactions), stabilizing the crystal-like state and therefore increasing the melting temperature and making the membrane less fluid. 1 Membrane Composition Influences Membrane Fluidity: Fatty Acid Structure unsaturated fatty acid chains saturated fatty acid chains lower Tm higher Tm OH OH O O 17 carbons cis double bond olive oil candle wax oleic acid stearic acid The degree of saturation of the fatty acid chains also affects the melting temperature and membrane fluidity. Fatty acids that are saturated, containing no double bonds, are straight and can pack more tightly than those that have double bonds. The kinks in the unsaturated chains simply make it more difficult to pack them in an orderly manner. As a consequence, the melting temperature of bilayers containing lipids with saturated fatty acids is higher than the melting temperature of bilayers containing lipids with unsaturated fatty acids. Additionally, the fluidity of bilayers containing lipids rich in unsaturated fatty acids is greater than the fluidity of bilayers containing lipids rich in saturated fatty acids. An example of this difference in fluidity and melting temperature is oleic and stearic acid. These two fatty acids have the same number of carbons (17), but they differ dramatically in their fluidity and melting temperature. At room temperature oleic acid is fluid-like (olive oil) and stearic acid is solid-like (candle wax); the melting temperature of oleic acid is lower than that of stearic acid because oleic acid is unsaturated and cannot pack in as orderly a manner as saturated stearic acid. 2 Membrane Composition Influences Membrane Fluidity: Cholesterol Content HO H H H H H Cholesterol is a major component of eukaryotic cells. The ratio of cholesterol to lipid molecules in a membrane can be as high as 1:1. Cholesterol is a member of a class of natural products called steroids, characterized by a 4 ring structure. Although all steroids are based on the same scaffold, they can affect different biological processes, ranging from the development of secondary sex characteristics (testosterone and estrogen) to inflammation (corticosteroids). Like phospholipids, cholesterol is an amphipathic molecule; it has a polar head that contains a hydroxyl group. The rest of cholesterol is hydrophobic, consisting of a rigid 4 ring steroid structure and a hydrocarbon tail. The 4 ring structure makes most of the cholesterol molecule very rigid because bonds between atoms in a ring are not free to rotate as a result of the geometric constraints of being in a ring. 3 Cholesterol Influences Membrane Fluidity Cholesterol interacts with phospholipids by orienting its polar hydroxyl head group close to the polar lipid head group. The rigid rings of cholesterol interact with and partly immobilize the fatty acid chains closest to the polar phospholipid head group. As a consequence, lipid molecules adjacent to cholesterol are less free to adopt different conformations than those in a cholesterol-free membrane region. By decreasing the mobility of a few methylene groups (CH2) in the fatty acids tails, cholesterol makes lipid bilayers less deformable and lessens their permeability to small water-soluble molecules. Therefore, cholesterol makes membranes less fluid. Although cholesterol makes bilayers less fluid, at the high concentrations of cholesterol found in eukaryotic cells, it also prevents fatty acid hydrocarbon chains from coming together and crystallizing. Therefore, cholesterol prevents fatty acid chains from ordering into a crystal- like state. Cholesterol inhibits phase transitions in lipids. At low temperatures it increases membrane fluidity by preventing fatty acid hydrocarbon chains from coming together and crystallizing. Under these conditions cholesterol inhibits the transition from liquid to solid (decreases the membrane freezing point). At high temperatures cholesterol decreases membrane fluidity by immobilizing a few methylene groups in the fatty acid tails of the lipids. Therefore, under these conditions cholesterol increases the melting point. Therefore, cholesterol acts like antifreeze - the temperature of your car engine is modulated by water circulating with antifreeze/coolant which lowers the freezing point of the antifreeze/coolant so it does not freeze in the winter. The antifreeze/coolant also raises the boiling point in the summer so that your engine does not overheat. The influence of cholesterol on membrane properties is critical for the normal functioning of eukaryotic cells. 4 Summary of Main Points • HIV needs host cells to replicate because its genome does not encode all of the proteins required for living systems • HIV recognizes host cells by interacting with specific protein receptors found on the surface of those cell types • Cell membranes are bilayers composed of amphipathic phospholipids containing charged head groups and hydrophobic tails • The hydrophobic effect drives the packing of lipids into structures which minimize exposed hydrophobic groups • Membranes are fluid because phospholipids and proteins can move in the plane of the bilayer; fatty acid structure and cholesterol content influence fluidity 5 October 19, 2006 6 Membrane Proteins and Membrane Transport 1. Membrane proteins a. Association of proteins with membranes b. Transmembrane helices 2. Lipid Rafts 3. Membrane transport a. Membrane permeability b. Transport proteins c. Ion distribution inside and outside cells d. Electrochemical gradient e. Active transport f. Ion channels 4. Membrane potential Lecture Readings Alberts 389-410 7 Membrane Proteins Although the lipid bilayer serves as a permeability barrier and provides the basic structure of all cell membranes, most membrane functions are carried out by membrane proteins, which constitute 50% of the mass of most plasma membranes. Membrane proteins perform many different functions: (1) they can transport ions and metabolites across the membrane (2) anchor the membrane to other proteins that play a roll in cell shape and structure (3) they can function as receptors to detect chemical signals in the environment and relay them to the inside of the cell (4) or they can act as enzymes to catalyze reactions. 8 Proteins Associate with Membranes in Different Ways Proteins can associate with the lipid bilayer in various ways: (a) Transmembrane proteins - These proteins extend through the bilayer and have some mass on both sides. They have hydrophobic sequences in the bilayer, interacting with the hydrophobic tails of lipids, and hydrophilic regions exposed to the aqueous environment on either side of the membrane. (b) Membrane associated - These are proteins located in the cytosol associated with the inner leaflet by an amphipathic alpha-helix (one side of the helix projects hydrophobic residues and the other side projects hydrophilic ones). (c) Lipid-linked - Some proteins lie entirely outside the bilayer, interacting with it using only a covalently attached lipid group. (d) Protein attached - Other proteins are associated only indirectly with the membrane, held there by interactions with one or more membrane proteins. 9 Many Membrane Proteins Cross the Bilayer Using One or More α-Helices Many proteins that have mass on either side of bilayer cross it using alpha-helices. Helical structures are are well-suited for folding in the bilayer. Those that are transmembrane have hydrophobic side chains which can interact with lipid tails. Within membranes, hydrogen bonding in the polypeptide backbone is maximized because water is absent from the bilayer. As a consequence, there is no competition with water for hydrogen bonding. ~20 amino acids are required to traverse the bilayer in an alpha-helical structure. Scientists can take advantage of knowledge of helical propensity of certain amino acids (the preference they have for or against being in an alpha-helical structure) and hydrophobicity to predict transmembrane domains in proteins. 10 Lipid Rafts in the Plasma Membrane protein with fatty protein with longer acid modification transmembrane region lipid bilayer enriched in cholesterol and phospholipids with long fatty acid tails As we saw in the animation, membranes are dynamic and are not uniform. Lipid rafts are a major source of inhomogeneity in the membranes of eukaryotic cells. Lipid rafts are microdomains in the lipid bilayer that are established by attractive
Details
-
File Typepdf
-
Upload Time-
-
Content LanguagesEnglish
-
Upload UserAnonymous/Not logged-in
-
File Pages24 Page
-
File Size-